You are here: Home > Section on DNA Replication, Repair, and Mutagenesis
Studies on DNA Replication, Repair, and Mutagenesis in Eukaryotic and Prokaryotic Cells
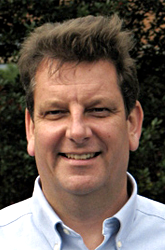
- Roger Woodgate, PhD, Head, Section on DNA Replication, Repair, and Mutagenesis
- Ekaterina Chumakov, PhD, Staff Scientist
- Elena Curti, PhD, Visiting Fellow
- Kiyonobu Karata, PhD, Visiting Fellow
- Wojciech Kuban, PhD, Visiting Fellow
- John McDonald, PhD, Biologist
- Justyna McIntyre, PhD, Visting Fellow
- Mary McLenigan, BS, Chemist
- Catherine Theisen, Student Fellow
- Alexandra Vaisman, PhD, Senior Research Fellow
Under optimal conditions, the fidelity of DNA replication is extremely high. Indeed, it is estimated that, on average, only one error occurs for every 10 billion bases replicated. However, given that living organisms are continually subjected to a variety of endogenous and exogenous DNA-damaging agents, optimal conditions rarely prevail in vivo. While all organisms have evolved elaborate repair pathways to deal with such damage, the pathways rarely operate with 100% efficiency. Thus, the persisting DNA lesions are replicated, but with much lower fidelity than is undamaged DNA. Our aim is to understand the molecular mechanisms by which mutations are introduced into damaged DNA. The process, commonly referred to as translesion synthesis (TLS) or translesion replication (TR), is facilitated by one or more members of the Y-family of DNA polymerases that are conserved from bacteria to humans. Based on phylogenetic relationships, Y-family polymerases may be broadly classified into five subfamilies; DinB-like (polIV/pol kappa-like) proteins are ubiquitous and found in all domains of life; in contrast, the Rev1-like, Rad30A (pol eta)-like, and Rad30B (pol iota)-like polymerases are found only in eukaryotes and the UmuC (polV)-like polymerases only in prokaryotes. We continue to investigate TLS in all three domains of life: bacteria, archaea, and eukaryotes.
Mutagenesis in prokaryotes
Most damage-induced (SOS) mutagenesis in Escherichia coli occurs when DNA polymerase V, activated by a RecA nucleoprotein filament (RecA*), catalyzes TLS. The biological functions of RecA* in homologous recombination and in mediating LexA and UmuD cleavage during the SOS response are well understood. In contrast, the biochemical role of RecA* in pol V–dependent mutagenic TLS remains poorly characterized. Proposals for the role of RecA* in TLS have evolved from positioning UmuD′C on primer/template DNA proximal to a lesion, to a dynamic interaction involving displacement of RecA* filaments on the template by an advancing pol V, to a model in which RecA* need not be located in cis on the template strand being copied, but can instead assemble on a separate ssDNA strand to transactivate pol V for TLS. As part of a collaborative study with Myron Goodman, we addressed the hitherto enigmatic role of RecA* in polV-dependent SOS mutagenesis. We demonstrated that RecA* transfers a single RecA•ATP stoichiometrically from its DNA 3′ end to free pol V (UmuD′2C) to form an active mutasome (pol VMut) with the composition UmuD′C•RecA•ATP. Pol VMut catalyzes TLS in the absence of RecA* and deactivates rapidly upon dissociation from DNA. Deactivation occurs more slowly in the absence of DNA synthesis, while retaining RecA•ATP in the complex. Reactivation of pol VMut is triggered by replacement of RecA•ATP from RecA*. Thus, the principal role of RecA* in SOS mutagenesis is to transfer RecA•ATP to pol V, so as to generate active mutasomal complex for translesion synthesis.
Development of high-throughput replication assays to study functions of specialized eukaryotic DNA polymerases
Human cells posses at least 14 DNA polymerases (pols). Three, pol alpha, pol delta, and pol epsilon are involved in genome duplication. The remaining eleven DNA polymerases have specialized functions within the cell. Four of the specialized DNA polymerases (pol eta, pol iota, pol kappa, and Rev1) belong to the Y-family of DNA polymerases and participate in TLS. Unlike cellular replicases, which are endowed with high processivity, high catalytic efficiency, and high fidelity, Y-family TLS DNA polymerases exhibit low processivity, low catalytic efficiency, and low fidelity. To facilitate our ongoing studies of the enzymology and cellular roles of these polymerases, a robust and flexible method for monitoring their catalytic activity was needed. In a collaborative study with Anton Simeonov’s group, we developed a fluorescence-based assay to study the enzymology of TLS DNA polymerases in real time. The assay is based on a fluorescent reporter strand displacement from a tripartite substrate containing a quencher-labeled template strand, an unlabeled primer, and a fluorophore-labeled reporter. With this assay, we could follow the activity of human DNA polymerases eta, iota, and kappa under various reaction conditions. We demonstrated that the assay can be used for small-molecule inhibitor discovery and investigation in highly miniaturized settings; we reported the first nanomolar inhibitors of Y-family DNA polymerases iota and eta. The fluorogenic replication assays we developed should facilitate further mechanistic and inhibitor investigations of eukaryotic TLS DNA polymerases.
Development of a DNA substrate to investigate the role of replicative clamps in TLS
We study the mechanisms by which mutations are introduced into damaged DNA. It is now known that many of the proteins long implicated in the mutagenic process are, in fact, low-fidelity DNA polymerases that can replicate by traversing damaged DNA by the TLS. The TLS polymerases gain access to a nascent primer terminus via an interaction with the cell’s replicative, ring-shaped, clamp (beta-clamp in E. coli and PCNA in eukaryotes). The process is initiated by a clamp loader (gamma-complex in E. coli and replication factor C in eukaryotes), which recognizes the DNA primer terminus and opens and assembles the clamp around the nascent DNA. Each clamp has two (prokaryotes)—or three (eukaryotes)—potential DNA polymerase–binding sites and may, therefore, engage multiple polymerases simultaneously. Indeed, such interactions are believed to be critical for switching between replicative and TLS polymerases.
In vitro studies investigating the effects of the replicative clamps on TLS have been hampered because the clamps readily slide off of linear DNA substrates. One option is to cap the DNA ends using large biomolecules such as streptavidin beads linked to biotinylated oligonucleotides. However, this imposes large steric constraints and may affect the ability of the DNA polymerase to access the primer terminus. Circular, single-stranded templates are, therefore, likely to provide more informative data on the effects of the replicative clamps on TLS and polymerase switching in vitro.
We therefore developed a protocol for the rapid and efficient purification of circular, single-stranded DNA containing a defined lesion. To achieve our goal, we used a primer containing a site-specific DNA lesion and annealed it to a single-stranded DNA template containing uracil. After primer extension and ligation, the double-stranded DNA is degraded in vitro, using the combined actions of E. coli uracil DNA glycosylase and exonucleases I and III. The final product is a circular, single-stranded DNA molecule containing a defined lesion that can be used for in vitro replication and repair assays involving replicative clamps.
Publications
- Curti E, McDonald JP, Mead S, Woodgate R. DNA polymerase switching: effects on spontaneous mutagenesis in Escherichia coli. Mol Microbiol 2009 71:315-331.
- Vidal AE, Woodgate R. Insights into the cellular role of enigmatic DNA polymerase iota. DNA Repair 2009 8:420-423.
- Karata K, Vidal AE, Woodgate R. Construction of a circular single-stranded DNA template containing a defined lesion. DNA Repair 2009 8:852-856.
- Jiang Q , Karata K, Woodgate R, Cox MM, Goodman MF. The active form of DNA polymerase V is UmuD’(2)CRecA-ATP. Nature 2009 460:359-363.
- Dorjsuren D, Wilson DM 3rd, Beard WA, McDonald JP, Austin CP, Woodgate R, Wilson SH, Simeonov A. A real-time fluorescence method for enzymatic characterization of specialized human DNA polymerases. Nucleic Acids Res 2009 [E-pub ahead of print].
Collaborators
- Myron F. Goodman, PhD, University of Southern California, Los Angeles, CA
- Anton Simeonov, PhD, NIH Chemical Genomics Center, NHGRI, Bethesda MD
- Antonio E. Vidal, PhD, Instituto de Parasitología y Biomedicina, Granada, Spain
- Samuel H. Wilson, MD, Laboratory of Structural Biology, NIEHS, Research Triangle Park, NC
Contact
For more information, email woodgate@mail.nih.gov or visit sdrrm.nichd.nih.gov.