You are here: Home > Section on Nervous System Development and Plasticity
Nervous System Development and Plasticity
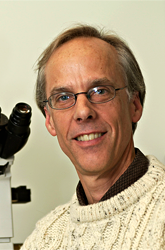
- R. Douglas Fields, PhD, Head, Section on Nervous System Development and Plasticity
- Olena Bukalo, PhD, Postdoctoral Fellow
- Philip Lee, PhD, Research Fellow
- Peter Wadeson, BS, Laboratory Technician
- Hiro Wake, MD, PhD, Visiting Fellow
Our research concerns molecular and cellular mechanisms by which functional activity in the brain regulates development of the nervous system during late stages of fetal development and early postnatal life. Areas of study include interactions between neurons and glia; the mechanisms of learning and memory; effects of neural impulses on cell proliferation, differentiation, neurite outgrowth, synaptogenesis, synapse plasticity, and myelination; and the regulation of gene expression in neurons and glia by specific patterns of neural impulses.
Activity-dependent neuron-glia interactions: myelin regulation by neural impulse activity
The importance of neural impulse activity in regulating the development of neurons is widely appreciated, but such is not the case for the development of non-neuronal cells in the brain (glia). With little attention historically focused on activity-dependent neuron-glia interactions outside the synapse, our laboratory is working to identify the mechanisms and functional significance of activity-dependent communication between axons and glia.
Myelin is the spiral wrapping of membrane around axons that provides electrical insulation essential for rapid impulse conduction. Our research suggests that myelin participates in cognition, psychiatric disorders, and learning by affecting the speed and synchrony of neural impulse transmission between cortical regions. Our findings show that myelinating glia can detect electrical activity in axons and that such detection influences glial development and myelination. We have identified several molecular mechanisms that enable myelinating glia to sense impulse activity in axons and to respond with changes in cell proliferation, differentiation, and myelination. These findings are relevant to early childhood development and offer new approaches to treating a demyelinating disease such as multiple sclerosis. At the same time, our work expands current concepts of activity-dependent plasticity and learning in the brain to include non-synaptic and non-neuronal communication.
We have shown that ATP (adenosine triphosphate) is released from axons firing action potentials and that the release activates purinergic receptors on myelinating glia, causing an increase in intracellular calcium, activation of transcription factors, regulation of gene expression, and control of glial development, cell proliferation, and myelination. In the central nervous system (CNS), we find that impulse activity promotes myelination. Adenosine, derived from the breakdown of ATP released from electrically active axons, stimulates differentiation of oligodendrocyte progenitor cells (OPC) to a promyelinating stage and promotes myelination by acting on P1 receptors. After OPCs mature, electrical activity can increase myelination in a different manner, which is dependent on ATP stimulation of another type of glial cell—the astrocytes—to release the cytokine LIF (leukemia inhibitory factor) that promotes myelination by mature oligodendrocytes. Our research shows that, in the peripheral nervous system (PNS), ATP released from axons firing action potentials inhibits Schwann cell proliferation, arresting cellular differentiation at an immature stage and inhibiting myelination. Our cellular and electron microscopic studies on LIF knockout mice show impairment of astrocyte proliferation and differentiation and reduced myelination.
Neuron-glia signaling in astrocyte development and plasticity
In the early postnatal hippocampus, glial progenitor cells proliferate and differentiate into astrocytes. This developmental process is correlated with the onset of spontaneous neural activity and may be relevant to how early experience can affect development of the brain. Our work shows that bidirectional signaling between neurons and glial cells contributes to the activity-dependent regulation of astrocyte development and in turn affects neuronal development, synaptogenesis, and synaptic function. Purinergic signaling (via extracellular ATP) has broad effects on both proliferation and differentiation of glial progenitor cells. We have found that ATP can be released from neurons following action potential firing and from astrocytes in response to neuronal activity; however, the mechanisms of ATP release from neurons and astrocytes are unclear. We are investigating the underlying mechanism of ATP release from axons and the response of astrocytes, oligodendrocytes, Schwann cells, and microglia and the subsequent effects on neuronal development and function. Recent findings indicate that interactions between purinergic and cytokine signaling regulate hippocampal glial and neuronal development in an activity-dependent manner, with effects on long-term potentiation of synaptic strength in the hippocampus. Similar processes also regulate synaptic development and function in the PNS at synapses with muscle (neuromuscular junction).
Hippocampal synaptic plasticity
It is widely appreciated that there are two types of memory: short-term and long-term. It has been known for decades that gene expression is necessary for converting short-term into long-term memory, but it is not known how signals reach the nucleus to initiate this process or which genes make memories permanent. Long-term potentiation (LTP) and long-term depression (LTD) are two widely studied forms of synaptic plasticity that can be recorded electrophysiologically in the hippocampus, and these phenomena are believed to represent a cellular basis for memory. We use cDNA microarrays to investigate the signaling pathways, genes, and proteins involved in LTP and LTD. We work to understand how regulatory networks are controlled by the appropriate patterns of impulses leading to different forms of synaptic plasticity and to identify new molecular mechanisms regulating synaptic strength.
We have identified sets of transcription factors, structural genes, and signaling pathways that are regulated by activity patterns leading selectively to different types of synaptic plasticity. One finding of particular interest is that expression of the gene BDNF, which encodes a growth factor, depends on whether or not firing of postsynaptic CA1 neurons is coincident with excitatory synaptic firing from presynaptic neurons.
Regulation of gene expression by action potential firing patterns
All information in the nervous system is encoded in the pattern of neural impulse activity. Given that experience regulates nervous system structure and function, gene activity in neurons must be regulated by the pattern of neural impulse activity. We tested this hypothesis by using custom cDNA arrays for gene expression profiling. We stimulated nerve cells to fire impulses in different patterns by delivering electrical stimulation through platinum electrodes in specially designed cell culture dishes. After stimulation, we measured mRNA and protein expression by gene arrays, quantitative RT-PCR (reverse transcriptase–polymerase chain reaction), Western blot, and immunocytochemistry. The results confirm our hypothesis that precise patterns of impulse activity can turn specific genes on or off. The experiments reveal signaling pathways and gene-regulatory networks that respond selectively to appropriate temporal patterns of action potential firing in neurons. Temporal aspects of intracellular calcium signaling are particularly important in regulating gene expression according to neural impulse firing patterns in normal and pathological conditions. We are also analyzing the role of post-transcriptional gene regulation mediated by mRNA stability and transport in hippocampal and DRG (dorsal root ganglion) neurons. Our findings provide a deeper understanding of how nervous system development and plasticity may be regulated by information coded in the temporal pattern of impulse firing in the brain.
Additional Funding
- Japanese Society for the Promotion of Science
Publications
- Fields RD. White matter in learning, cognition, and psychiatric disorders. Trends Neurosci 2008 31:361-370.
- Fields RD. White matter matters. Sci Am 2008 298:54-56.
- Fields RD. Oligodendrocytes changing the rules: action potentials in glia and oligodendrocytes controlling action potentials. Neuroscientist 2008 14:540-543.
- Ishibashi T, Lee PR, Baba H, Fields, RD. Leukemia inhibitory factor regulates the timing of oligodendrocyte development and myelination in the postatal optic nerve. J Neurosci Res 2009 87:3343-3355.
- Cohen JE, Fields RD. Activity-dependent neuron-glial signaling by ATP and leukemia-inhibitory factor promotes hippocampal glial cell development. Neuron Glia Biol 2008 4:43-55.
Collaborator
- Tomoko Ishibashi, PhD, Tokyo University of Pharmacy and Life Science, Tokyo, Japan
Contact
For more information, email fieldsd@mail.nih.gov or visit nsdps.nichd.nih.gov