You are here: Home > Microscopy and Imaging Core Facility
Microscopy and Imaging Core Facility
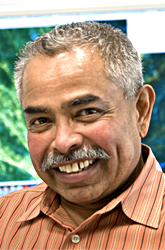
- James T. Russell, DVM, PhD, Director
- Louis (Chip) Dye, BS, Staff Scientist
- Vincent Schram, PhD, Staff Scientist
- Lynne A. Holtzclaw, BS, Senior Research Assistant (Biologist)
The mission of the NICHD Microscopy and Imaging Core (MIC) is to provide high-end light and electron microscopy services to all NICHD scientists. The Core is designed as a multi-user facility where investigators can, with a minimum expense of time and effort, prepare, image, and analyze their samples. Located in the 5th and 6th floors of building 49, the facility is staffed with three full-time microscopists working under the supervision of James Russell. Vincent Schram oversees the light-microscopy operations and IT infrastructure, Lynne Holtzclaw supports light microscopy and immunohistochemistry, and Louis (Chip) Dye manages the electron microscopy arm of the facility.
Mode of operation
The equipment and staff of the MIC are available to everyone within the Institute. The philosophy of the MIC is to ensure that only reliable, high-quality data are recorded on its instruments. For every new project, MIC staff meet with the Principal Investigator and the postdoctoral scientists involved in the study to discuss details of the experimental design. The background of the project and imaging goals are discussed at that time, and the most appropriate techniques and instrumentation are determined. Users are asked to sign a document outlining the policies to follow when using the Core equipment (www.nichd.nih.gov/about/org/dir/other-facilities/cores/microscopyandimaging/policies). Positive feedback from long-term users suggests that this high level of interaction greatly improves the quality of data obtained and efficiency of each imaging project. The facility is accessible 24/7 using the NIH ID system, and users can reserve time on each instrument by using an online calendar (next.cirklo.org/nichd).
The MIC implemented a fee structure starting the past year. The fee-for-service model was approved by the Program Heads through a comment process during the summer of 2010. As of this writing, the rates for NICHD researchers are between $20 to $30 per hour for confocal imaging. Other Institutes were charged at a slightly higher rate, but so far we have not billed other institutes due to very modest usage and the difficulty of transferring funds between Institutes.
Light microscopy
The light microscopy arm of the MIC operates in four different areas:
Equipment maintenance and upgrade. The MIC operates several confocal laser scanning microscopes optimized for different applications: Zeiss LSM 510 inverted for high-resolution confocal imaging of fixed specimen, Zeiss Live DuoScan for high-speed imaging of live cells, and a Zeiss LSM 510 NLO for two-photon imaging of live tissue sections and live animals. The aging Perkin-Elmer spinning disk instrument for low-light imaging of photo-sensitive specimens was decommissioned during the past year because of severe degradation in image quality and sensitivity. Two sub-resolution instruments, a dual-channel total internal reflection fluorescence (TIRF) Olympus platform and a home-built photo-activation localization microscope (PALM), are available. A high-end wide-field fluorescence instrument and a fluorescence stereo microscope round out MICs equipment portfolio.
Live imaging is supported with temperature, CO2, and humidity control and heated perfusion on most microscopes. Instrument downtime is kept to a minimum by providing full-time support to end users (phone and pager). For problems that require extensive repairs, most instruments are covered by manufacturers' service contracts and are usually serviced within a few days.
User training and support. After counseling on specimen preparation and staining, each user receives hands-on training on the light microscope required for the project. The training covers the principles of fluorescence microscopy, confocal imaging, and optimum operation of the hardware platform and is followed by periodic refreshers at the user's request or when the MIC staff feels the equipment is not being used optimally.
Image analysis. The MIC operates a data analysis center with three high-end workstations and imaging software (Metamorph, Volocity, Imaris and Zeiss AIM). At the users' request, we provide training and support for each software package and, when required, custom macros and high-throughput image analysis solutions. The facility offers extensive data storage services with an enterprise-level file server and a data backup system. This infrastructure is used to safeguard images and move data from the facility to each user's location on campus.
Methods development. During the past year, Vincent Schram completed installation of a fluorescence PALM (FPALM) platform. The image acquisition and analysis software modules are now complete for single-color imaging. Similar method-development efforts will be taken on according to Institute needs and availability of funds. We actively entertain such requests.
Electron microscopy
Because of the complex and delicate nature of electron microscopy (EM) sample preparation, the EM arm of the MIC operates differently from the light microscopy imaging side.
Sample processing. Typically, all EM processing (fixation, embedding, cutting, and staining) is undertaken in-house by Chip Dye. The MIC features a fully equipped EM laboratory with a LKB Pyramitome, a Leica CM3050-S Cryostat, and a Reichert Ultracut-E Ultramicrotome. Owing to the labor involved, fewer projects are undertaken. By providing consistent and controlled incubation parameters, the PELCO Biowave Pro programmable incubator (Ted Pella, Inc.) has been instrumental in improving the preservation of ultrastructure and the quality of immunolabeling.
Imaging. EM imaging is done most of the time by the microscopist, except in cases where the user has the necessary inclination and training. During the past year, the MIC replaced its aging JEOL 1010 transmission electron microscope with a modern JEOL 1400 series instrument. The scope was installed during the Spring of 2011 and is currently operational. It offers two new imaging methodologies: cryo-EM and tomography. The main advantage of cryo-EM is to preserve a high level of immunoreactivity and allow specimens to be imaged in a near native state. Cryo-EM will improve the quality of studies relying on immunogold labeling. Tomography provides three-dimensional imaging of structures at the EM level.
Methods development. Chip Dye has set up techniques for EM-level immunohistochemistry and double immunolabeling to simultaneously label two separate antigens and is using LUXFilm EM grids, which provide a view of the entire specimen and are crucial for imaging large structures, tracing features, searching for special details, and tomography imaging. The MIC also implemented a digital archive of all EM images and parameters, which will be available online to investigators in the near future.
Ancillary support
Given that the NICHD's Division of Intramural Research laboratories are scattered all over the NIH campus, the MIC provides, within a single building, all necessary techniques and facilities, such as tissue culture hood, 5 and 10% CO2 incubators, animal holding and preparative space, and vibratomes for live and fixed tissues. Lynne Holtzclaw provides outstanding technical expertise on advanced cell and tissue-sample preparation for immunohistochemistry experiments.
Community outreach
The MIC is committed to promoting light and electron microscopy in the NICHD, DIR research community. We are making efforts to educate investigators on the benefits and pitfalls of advanced imaging techniques. These initiatives include (i) coaching users on the principles of confocal microscopy, during training and via publication of comprehensive operating protocols for each microscope; (ii) on-campus demonstrations of new instruments and software by vendors such as Zeiss, Olympus, Photometrics, Nikon and Perkin-Elmer; and (iii) on-site assistance to investigators in their laboratories operating their own imaging equipment to optimize the quality of the data recorded. Furthermore, the MIC website (mic.nichd.nih.gov) is an important resource for tutorials and protocols for both fixed and live cell microscopy.
Parallel to these efforts, the staff have continued to collaborate with other Institutes to promote the exchange of information and bring new imaging technologies to this Institute. Ongoing collaborations include: imaging of live animals (NINDS); sub-resolution light microscopy (F-PALM) with the trans-NIH Imaging initiative; and software development efforts with the Center for Information Technology.
Facility usage
The MIC currently serves a total of 125 registered users associated with 41 NICHD principal investigators (PIs) and three PIs of sister Institutes within the campus. On any given week, approximately 10 different users spend half a day or more on an MIC microscope. As of October 2011, users have logged more than 38,000 hours on the Core's equipment. Since its creation in 2004, usage has resulted in more than 78 publications, 7 of which have been co-authored by Core personnel (see www.nichd.nih.gov/about/org/dir/other-facilities/cores/microscopyandimaging/publications for a complete list).
Looking ahead
The MIC continues to suffer from its aging equipment portfolio. Ninety percent of the light imaging platforms were purchased between 2003 and 2005 and no longer provide the level of performance required in today's competitive publishing environment. A proposal to replace the most used point-scanning-confocal microscope (a Zeiss LSM 780) was recently approved, and the instrument has been ordered. As stated earlier, the JEOL transmission electron microscope was replaced last spring with a modern platform allowing for tomography and cryo-EM. MIC would still require additional equipment (high-pressure freezing unit, cryo-ultra-microtome, and a freeze substitution unit) in order to make CyroEM technology routinely available to investigators. The MIC continues its efforts towards technology development. The sub-resolution FPALM platform was completed during the last year. Similarly, the EM arm of MIC completed development of methods for multiple immuno-labeling. The new JEOL 1400 instrument will significantly improve the EM capabilities of the Core by providing cryo-EM and tomography.
The portfolio of instruments within the MIC needs to be maintained at peak levels of performance at all times to serve the needs of NICHD scientists adequately. The quality of data acquired is heavily influenced by the quality of the optics. This can be achieved only with timely upgrades and replacement of aging instruments. The fee-for-use model adversely impacted instrument usage, while failing to generate significant revenues for the MIC. The past year clearly showed that fees provide a supplement rather than an alternative to the DIR's support. If the MIC is expected to provide high-end imaging solutions to the DIR, a new support model that allows for timely equipment upgrades is critical.
Scientific activities within the MIC: characterization of signaling between axons and Schwann cells that myelinate them
Glial cells monitor and respond to neural activity by conditioning the extacellular milieu and by signaling within glial cell networks, as well as by sending signals back to neurons. Unlike neurons, which use electrical signals to communicate, glial cells possess a form of Ca2+-based excitability by which, in response to synaptic activity, they generate and propagate intracellular Ca2+ signals as waves over long distances. This intimate communication between glial cells and neurons is crucial for normal brain development and appears to play a critical role in the plastic functions of the brain. We aim to understand the nature of these signals in glial cells.
In collaboration with James Pickel, we generated transgenic mouse lines expressing YC3.60, a fluorescent Ca2+ indicator protein, which, by using the human S100β promoter sequence, was expressed only in astrocytes in the brain and in Schwann cells in peripheral nerves. We used two-photon confocal microscopy to image cellular Ca2+ signals in astrocytes or Schwann cells in brain slice preparations, anesthetized intact mice, or isolated sciatic nerve preparations, permitting an investigation of Schwann-cell Ca2+ signals in response to action potential propagation in axons.
We had shown earlier that proteins involved in Ca2+ signaling are concentrated in regions of the Schwann cell around nodes of Ranvier in the sciatic nerve. We found the paranodal region and the juxtaparanode to be rich in ion channels involved in metabotropic Ca2+ signaling, suggesting that specialized cellular regions may initiate Schwann cell signals during action potential propagation. To test this hypothesis, we used two-photon confocal microscopy to image sciatic nerves isolated from transgenic mice expressing YC3.60 in Schwann cells. We stimulated the nerve bundles with a suction electrode and recorded compound action potentials during stimulation. Action potentials elicited by electrical stimulation induced robust Ca2+ rises in Schwann cells, particularly in the paranodal region. The action potential–dependent Schwann cell Ca2+ response was abolished in the presence of purinergic antagonists that are specific for the P2Y2 subtype of receptor.
Application of exogenous purinergic agonists to isolated sciatic nerve axons readily elicited Ca2+ signals in Schwann cells, as revealed by YC3.60 fluorescence changes. The rank order of potency of purinergic agonists was ATP>UTP>2MeSATP, suggesting the presence of P2Y-subtype purinergic receptors on Schwann cells. Furthermore, these purinergic agonist–elicited Ca2+ signals persisted in the complete absence of Ca2+ ions in the extracellular medium, suggesting that P2X-type purinergic receptors did not contribute to the signal. In support of this conclusion, prolonged exposure to UTP to deplete intracellular Ca2+ stores, while abolishing a response to ATP, did not evoke a response to a P2X-selective purinergic agonist. The experiments showed that Schwann cells in situ express a functional P2Y-subtype of purinergic receptor, a novel finding, and it is likely that the receptor system is involved in Schwann-cell responses to acute nerve injury. It is well known that, following injury, Schwann cells retract, leading to initial demyelination, followed by regeneration. We also intend to investigate Schwann-cell signals associated with nerve injury. The transgenic mouse line expressing the Ca2+ indicator in Schwann cells provides, for the first time, a suitable experimental model for studying acute and chronic Schwann-cell signals associated with nerve injury.
Our current efforts are directed at understanding the mechanisms that support action potential–dependent communication between axons and Schwann cells. We are exploring the consequences of action potential traffic for ionic concentrations within the axon and extracellular milieu in the tight spaces between the two cells. The study is almost complete, and a manuscript is in preparation.
The strategy of directing expression of Ca2+ indicator photoproteins in a cell-specific manner has proven extremely valuable for investigating glial cells' physiological responses during nervous system function, both in isolated preparations and in situ, and we are continuing to exploit such powerful tools. In collaboration with James Pickel, we are developing glial plasmids targeting specific glial cell types. These new tools will be based on the latest indicators, which provide enhanced photo-stability and brightness. Furthermore, we are using novel promoters that both provide cell-type specificity and enhance indicator expression. We are now generating plasmids containing TN-XXL and GCAMP3, a novel class of indicators with the aforementioned advantages. In addition, we are using the astrocyte-specific Glt-1 gene promoter to target astrocytes and the cyclic nucleotide phosphodiesterase (CNP) promoter to target Schwann cells and OP cells.
References generated in 2011 with the assistance of the MIC
- Cea-del Rio CA, Lawrence JJ, Erdelyi F, Szabo G, McBain C.J. Cholinergic modulation amplifies the intrinsic oscillatory properties of CA1 hippocampal cholecystokinin-positive interneurons. J Physiol 2011:589:609-627.
- Huang KP, Huang FL, Shetty PK. Stimulation-mediated translocation of calmodulin and neurogranin from soma to dendrites of mouse hippocampal CA1 pyramidal neurons. Neuroscience 2011;178:1-12.
- Huang KP, Huang FL. Calcium-sensitive translocation of calmodulin and neurogranin between soma and dendrites of mouse hippocampal CA1 neurons. ACS Chem Neurosci 2011;2:223-230.
- Komlosh ME, Özarslan E, Lizak MJ, Horkay F, Schram V, Shemesh N, Cohen Y, Basser PJ. Pore diameter mapping using double pulsed-field gradient MRI and its validation using a novel glass capillary array phantom. J Magn Reson 2011;208:128-135.
- Li S, Bjelobaba I, Yan Z, Kucka M, Tomic M, Stojilkovic SS. Expression and roles of pannexins in ATP release in the pituitary gland. Endocrinology 2011;152:2342-2352.
- Liu M, Eiden MV. The receptors for gibbon ape leukemia virus and amphotropic murine leukemia virus are not downregulated in productively infected cells. Retrovirology 2011;8:53.
- Liu XH, Xu W, Russ J, Eiden LE, Eiden MV. The host range of gammaretroviruses and gammaretroviral vectors includes post-mitotic neural cells. PLoS One 2011;6:e18072.
- Neddens J, Fish KN, Tricoire L, Vullhorst D, Shamir A, Chung W, Lewis DA, McBain CJ, Buonanno A. Conserved interneuron-specific ErbB4 expression in frontal cortex of rodents, monkeys, and humans: implications for schizophrenia. Biol Psychiatry 2011;70:636-645.
- Nestor MW, Hoffman DA. Differential cycling rates of Kv4.2 channels in proximal and distal dendrites of hippocampal CA1 pyramidal neurons. Hippocampus 2011 [Epub ahead of print].
- Park JJ, Gondré-Lewis MC, Eiden LE, Loh YP. A distinct trans-Golgi network subcompartment for sorting of synaptic and granule proteins in neurons and neuroendocrine cells. J Cell Sci 2011;124: 735-744.
- Sarkar C, Zhang Z, Mukherjee AB. Stop codon read-through with PTC124 induces palmitoyl-protein thioesterase-1 activity, reduces thioester load and suppresses apoptosis. Mol Genet Metab 2011;104:338-345.
- Subramanian J, Morozov A. Erk1/2 inhibit synaptic vesicle exocytosis through L-type calcium channels. J Neurosci 2011;31:4755-4764.
- Tanaka NK, Dye L, Stopfer MJ. Dual-labeling method for electron microscopy to characterize synaptic connectivity using genetically encoded fluorescent reporters in Drosophila. J Neurosci Methods 2011;194:312-315.
- Tricoire L, Pelkey KA, Erkkila BE, Jeffries BW, Yuan X, McBain CJ. A blueprint for the spatiotemporal origins of mouse hippocampal interneuron diversity. J Neurosci 2011;31:10948-10970.
- Wang PM, Kachel DL, Cesta MF, Martin WJ. Direct leukocyte migration across pulmonary arterioles and venules into the perivascular interstitium of murine lungs during bleomycin injury and repair. Am J Pathol 2011;178:2560-2572.
- Wei H, Zhang Z, Saha A, Peng S, Chandra G, Quezado Z. Mukherjee AB. Disruption of adaptive energy metabolism and elevated ribosomal p-S6K1 levels contribute to INCL pathogenesis: partial rescue by resveratrol. Hum Mol Genet 2011;20:1111-1121.
Publications
- Russell JT. Imaging calcium signals in vivo: a powerful tool in physiology and pharmacology. Br J Pharmacol 2011;163:1605-1625.
- Jiang XS, Wassif CA, Backlund PS, Song L, Holtzclaw LA, Li Z, Yergey AL, Porter FD. Activation of Rho GTPases in Smith-Lemli-Opitz syndrome: pathophysiological and clinical implications. Hum Mol Genet 2010;19:1347-1357.
- Komlosh ME, Özarslan E, Lizak MJ, Horkay F, Schram V, Shemesh N, Cohen Y, Basser PJ. Pore diameter mapping using double pulsed-field gradient MRI and its validation using a novel glass capillary array phantom. J Magn Reson 2011;208:128-135.
- Tanaka NK, Dye L, Stopfer M. Dual-labeling method for electron microscopy to characterize synaptic connectivity using genetically encoded fluorescent reporters in Drosophila. J Neurosci Meth 2011;194:312-315.
Collaborators
- Ranjan Gupta, MD, PhD, University of California Irvine, Irvine, CA
- James Pickel, PhD, Laboratory of Genetics, NIMH, Bethesda, MD
- Afonso Silva, PhD, Cerebral Microcirculation Unit, NINDS, Bethesda, MD
Contact
For more information, visit mic.nichd.nih.gov.