You are here: Home > Bone and Extracellular Matrix Branch
Genetic Disorders of Bone and Extracellular Matrix
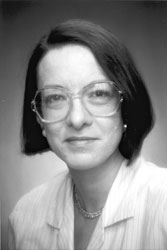
- Joan C. Marini, MD, PhD, Chief, Bone and Extracellular Matrix Branch
- Aileen M. Barnes, MS, Research Associate
- Wayne A. Cabral, AB, Chemist
- Simone M. Smith, PhD, Research Fellow
- Heeseog Kang, PhD, Postdoctoral Fellow
- Adi Reich, PhD, Postdoctoral Fellow
- Vihang V. Nakhate, BS, Postbaccalaureate
- Brandi M. Owens, BA, Postbaccalaureate
In an integrated program of laboratory and clinical investigation, the Bone and Extracellular Matrix Branch (BEMB) studies the molecular biology of the heritable connective tissue disorders osteogenesis imperfecta (OI) and Ehlers-Danlos syndrome (EDS). Our objective is to elucidate the mechanisms by which the primary gene defect causes skeletal fragility and other connective tissue symptoms and then to apply this knowledge to the treatment of children with these conditions. Recently, we identified the long-sought cause of recessive OI. Discoveries of defects in collagen modification generated a new paradigm for collagen-related disorders of matrix. We established that structural defects in collagen cause dominant OI while deficiency of proteins that interact with OI for folding, post-translational modification, or processing cause recessive OI. Our challenge now is to understand the cellular and biochemical mechanisms of recessive OI. We also generated a knockin murine model for OI with a classical collagen mutation as well as a murine model for recessive type IX OI and are using these models to study disease pathogenesis and the skeletal matrix of OI, the effects of pharmacological therapies, and approaches to gene therapy. Our clinical studies involve children with types II and IV OI, who form a longitudinal study group enrolled in age-appropriate clinical protocols for the treatment of their condition.
Mechanism of rare forms of osteogenesis imperfecta (OI)
Deficiency of components of the collagen prolyl 3-hydroxylation (P3H) complex causes recessive OI. Thus, deficiency in CRTAP (collagen-associated protein) causes type VII OI (#610862 in the OMIM database), while deficiency in P3H1 causes type VIII OI (OMIM #610915). The endoplasmic reticulum (ER)–resident 3-hydroxylation complex, of which cyclophilin B is the third member, is responsible for the 3-hydroxylation of the Pro986 residue of the α1(I) chains. Null mutations in LEPRE1, the gene encoding P3H1, or CRTAP cause a severe to lethal bone dysplasia whose phenotype overlaps with the severe/lethal dominant forms of OI in terms of crumpled and under-tubulated long bones, disorganized bone matrix, and extreme bone fragility. However, the two recessive forms share other characteristics that are distinct from those of dominant OI; affected individuals have white or light sclerae, small/normal rather than enlarged head circumferences, and rhizomelia of long bones. Biochemically, the type I collagen produced by affected children lacks Pro986 hydroxylation but, surprisingly, exhibits over-modification of the helical region of collagen chains by the P4H1 and LOH modification system, indicating that folding of the helix is delayed. We found that both CRTAP and P3H1 are absent from cells when either gene that encodes the proteins has a null defect, demonstrating mutual protection of these two components in the 3-hydroxylation complex.
Cyclophilin B (CyPB), encoded by PPIB, is an ER–resident peptidyl-prolyl cis-trans isomerase (PPIase) that functions both independently and as a component of the collagen prolyl 3-hydroxylation complex. CyPB is proposed to be the major PPIase catalyzing the rate-limiting step in collagen folding. Previously, the BEMB characterized the first patient with deficiency of PPIB, which causes recessively inherited Type IX OI. However, in contrast to P3H1 and CRTAP deficiency, type I collagen from the CyPB–deficient patient did not appear to be overmodified, suggesting redundancy for CyPB’s collagen isomerization function. To understand these unexpected observations, our group generated a Ppib knock-out (KO) mouse model that recapitulates the Type IX OI phenotype, including growth deficiency and brittle bones (5). Collagen from KO mouse tissue and cells is nearly lacking 3-hydroxylation.
Using tissues and cell lines derived from the Ppib KO mouse, we investigated the role of CyPB in collagen biosynthesis and found that intracellular collagen folding occurs more slowly in CyPB-null cells, supporting its role in the rate-limiting step of folding. However, treatment of KO cells with the cyclophilin inhibitor cyclosporin A caused further delay in folding, providing additional support for the existence of another collagen PPIase. We also extended the reported role of CyPB in supporting collagen lysyl hydroxylase (LH1) activity. As expected, the delayed rate of folding in the absence of CyPB alters hydroxylation and glycosylation of collagen lysyl residues. Importantly, analysis of bone and osteoblast type I collagen revealed site-specific alterations in helical lysine hydroxylation, in particular, significantly reduced hydroxylation of helical crosslinking residue K87. The alteration directly affects both the extent and type of collagen intermolecular crosslinks that form in bone tissue. Our studies demonstrated novel consequences of the effect of CyPB on collagen hydroxylation, glycosylation, crosslinking, and fibrillogenesis, showing that CyPB not only functions to regulate collagen folding and 3-hydroxylation in the ER but also indirectly regulates bone development and mechanical properties.
Investigations of the mechanism of type XI OI, caused by absence of the peptidylprolyl isomerase FKBP65, have been a new focus of our studies. FKBP65 is a collagen-binding ligand. Deficiency of FKBP65 causes progressive deforming OI, with or without congenital contractures. We demonstrated that type I collagen folding kinetics are normal in the absence of FKBP65, but that collagen cross-linking and deposition of collagen into matrix are strongly reduced, resulting in a matrix with sparse and disorganized collagen fibrils. The reduced collagen deposition is caused by near absence of collagen telopeptide hydroxylation in the proband collagen. The hydroxylation of collagen telopeptide lysine is catalyzed by LH2 (lysyl hydroxylase 2), which provided the first indication that FKBP65 is crucial for LH2 activity.
More recently, we extended the phenotype of FKBP10 mutations from OI and OI plus contractures to Kuskokwim syndrome (KS), a congenital contracture disorder. In KS patients, we identified a 3 nt deletion in FKBP10, which deletes a highly conserved residue in the third isomerase domain (1). FKBP65 is destabilized in this condition to 5% residual protein. Hydroxylation of the collagen telopeptide lysine is reduced to 2–10% in KS, suggesting an intermediate reduction of crosslinks. Other contracture syndromes may be caused by FKBP10 defects.
OI type V is caused by a recurrent dominant mutation (c.-14C→T) in IFITM5, which encodes BRIL, a bone-restricted interferon-induced transmembrane protein–like protein most strongly expressed in osteoblasts, which plays a role in mineralization. Patients with type V OI have distinctive clinical manifestations with overactive bone mineralization and mesh-like lamellation on bone histology. Recessive null mutations in SERPINF1, which encodes pigment epithelium–derived factor (PEDF), cause OI type VI, which impairs mineralization. Type VI OI patients have no serum PEDF, elevated alkaline phosphatase (ALPL) as children, and bone histology with broad unmineralized osteoid and fish-scale pattern. The two types of OI appear to be unconnected, caused by different genes, with distinct phenotypes and histology.
However, we identified a patient with severe OI whose phenotype is most like type VI OI. Her osteoblasts displayed minimal secretion of PEDF, but her serum PEDF was normal. SERPINF1 sequences were normal despite bone histomorphometry typical of type VI OI, and elevated childhood serum ALPL. To identify the mutation, exome sequencing on the proband, parents, and an unaffected sibling surprisingly yielded a de novo mutation in IFITM5 in one allele of the proband, causing a p.S40L substitution in the BRIL intracellular domain (4). IFITM5 transcript and BRIL protein level were normal in proband fibroblasts and osteoblasts. SERPINF1 expression and PEDF secretion were decreased in proband osteoblasts. In contrast, osteoblasts from a typical case of type V OI have elevated SERPINF1 expression and PEDF secretion during osteoblast differentiation. Together, the data suggest that BRIL and PEDF have a relationship that connects the genes for types V and VI OI and their roles in bone mineralization.
A collaboration with Bernd Wollnik led to the identification of WNT1 defects in OI that cause phenotypes ranging from severe bone fragility to early onset osteoporosis. Mutant WNT1 proteins fail to activate canonical LRP-5–mediated β-catenin signaling (LRP-5 stands for low-density lipoprotein receptor–related protein 5).
West African LEPRE1 allele for type VIII OI
Our initial studies of type VIII OI identified a recurring mutation, IVS5+1G→T, in African Americans and West African immigrants to the United States. The mutation was later identified in other individuals of African origin, but not in any other racial group. Homozygotes for the mutation die within months of birth, while compound heterozygotes can survive, but with extremely severe bone disease. We hypothesize that this is a West African founder mutation, which was transported to the Caribbean and the Americas with the Atlantic slave trade. To investigate the molecular anthropology of the mutation, we screened contemporary African Americans from the Middle Atlantic States, as well as contemporary Africans. In three African American cohorts, the carrier incidence was 1/200–300 individuals, typical of a rare disorder and predicting a frequency of homozygous type VIII OI among African Americans of about 1/200,000 births. For West Africans, we collaborated with Charles Rotimi, who shared over 1,200 DNA samples from contemporary Ghanaians and Nigerians. To our surprise, the carrier frequency for this lethal bone dysplasia in West Africa is over 1.5%. The particularly high carrier incidence predicts that the rate of recessive OI in West Africa will equal the rate of dominant OI. In collaboration with Sarah Tishkoff and Timothy Rebbeck, we determined that the mutation was not pan-African, but rather was limited to West Africa. Using haplotype analysis of African American pedigrees and West African triads, we estimate that the mutation first occurred about 600 years ago, prior to the Atlantic slave trade, consistent with our hypothesis.
C-propeptide cleavage site mutations increase bone mineralization.
Type I procollagen is processed to mature collagen by the removal of both N- and C-terminal propeptides. The C-propeptide is cleaved at the Ala-Asp peptide bond between the telopeptide and the C-propeptide of each chain by C-proteinase/BMP-1; cleavage is enhanced by C-proteinase enhancer protein (PCPE-1). Probands with substitutions at the four cleavage site residues have been identified; we are studying two of those probands (3). Surprisingly, the two children have elevated bone density DEXA z-scores, although their fracture history, radiographs, and bone histomorphometry are typical of mild OI. The only abnormality in collagen biochemistry in these children is delayed cleavage of the C-propeptide, which led us to investigate matrix mineralization.
In collaboration with Adele Boskey, we found that Fourier transform infrared spectroscopy (FTIR) demonstrated a higher mineral/matrix ratio in both the trabecular and cortical bone of each patient than in either age-matched normal or classical OI controls, as well as marked maturation of collagen cross-links, although crystallinity and mineral composition were typical of classical OI. We extended the investigation of mineralization with BMDD (bone mineral density distribution) and BEI (backscattered electron imaging) to show that, in the α2(I) cleavage site mutation, the bone had a uniformly higher mineral density, while in the α1(I) mutation, the average mineral density was typical of classical OI but markedly more heterogeneous, with areas of very high and low bone density.
Modeling of the collagen fibril indicated that the C-terminal end is located on the surface of the fibril. If the C-propeptide is not cleaved, its surface location on the fibril apparently generates an initiating locus for increased mineralization. The studies showed that mutations in the C-propeptide cleavage site cause a distinctive form of OI and revealed important basic information about the propeptide's role in bone mineralization. To study the developmental progression of a C-propeptide cleavage site mutation, we are developing a knock-in murine model with a COL1A1 mutation.
Insight from the Brtl mouse model for OI
The Brtl mouse model for OI, generated by the BEMB, is a knock-in mouse that contains a Gly349Cys substitution in the α1(I) chain. Brtl was modeled on a type IV OI child and accurately reproduces features of type IV OI. Brtl has provided important insights into both potential OI treatments and the mechanism of OI. First, we conducted a treatment trial of the bisphosphonate alendronate in Brtl and wild-type (WT) littermates. We found that bone density, bone volume, and trabecular number improved with treatment, as did load-to-fracture. However, detrimental side effects such as retained mineralized cartilage, reduced material properties, and altered osteoblast morphology occurred with treatment. The results reinforce the conclusion of the pediatric trial to limit the duration of bisphosphonate treatment. Collaborative studies with Kenneth Kozloff, using an ulnar loading model, demonstrated that Brtl mouse long bone is more susceptible to microdamage. The susceptibility may partially account for the decline in material quality when OI bone is treated with bisphosphonates, which inhibit the osteoclast activity necessary for micro-damage repair. Recently, we also collaborated with Kozloff's group to investigate a potential anabolic therapy, sclerostin antibody (Scl-Ab), which stimulates osteoblasts via the canonical wnt pathway. Scl-Ab stimulated bone formation in Brtl mice and increased bone mass and load-to-fracture. In treatment with Scl-Ab, there was no change in Brtl bone material properties. Nano-indentation studies indicating unchanged mineralization showed that the hypermineralization of bisphosphonate treatment did not occur.
A third therapeutic trial involving Brtl was in utero cell transplantation of normal mesenchymal stem cells (MSC) tagged with GFP. Surprisingly, despite the low-level of cell uptake into bone, the day-one lethality outcome for 30% of Brtl mice was almost entirely rescued, and the biomechanical properties of Brtl femora at two months were significantly improved. The results coincide with our previous data on human mosaic carriers who have less than 30% mutant bone cells and a mild phenotype.
Brtl also provided important information about the cellular function in OI. Comparison of lethal and surviving Brtl pups found that ER stress was significantly elevated in the lethal pups. Gadd153, also known as CHOP, a component of an apoptosis pathway, was elevtated in bone cells of lethal pups. Collaborative studies demonstrated impairment in the differentiation of Brtl MSCs to osteoblasts, including reduced expression of early and late osteoblastic markers. Conversely, Brtl MSCs generated more and larger adipocytes than WT MSCs. Other studies of surviving Brtl mice demonstrated the importance of osteoclasts to the OI phenotype. Osteoclast surface in Brtl is increased and is uncoupled from osteoblast surface, resulting in a decline in bone formation rate (BFR). Surprisingly, the number of TRAP+ (a marker for osteoclasts) cells is elevated in Brtl marrow cultures, suggesting elevated numbers of precursors. We demonstrated that this increase is independent of the RANKL/OPG signaling pathway and are currently exploring other osteoclast-stimulating pathways.
Co-culture experiments with Brtl and WT MSC's and osteoclast precursors yielded elevated osteoclast numbers from WT or Brtl precursors grown with Brtl MSCs, but not with WT MSCs. The results indicate that an osteoblast product is necessary and sufficient for elevated osteoclast numbers and could provide an important target for treatment of OI.
Natural history and bisphosphonate treatment of children with types III and IV OI
We recently brought the cardiopulmonary aspects of our natural history study on types III and IV OI to publication in collaboration with translational murine studies of our collaborator, Martin Hrabe de Angelis (2). The longitudinal evaluations were completed in 23 children with type III OI and 23 children with type IV OI, who had serial pulmonary function tests every 1–2 years. Comparison of their results with size-matched children showed a significant decline over time in pulmonary function, including lung volumes and flow rates. The decline was worse in the 36 children with scoliosis (average curve 25 degrees) but also occurred in 20 participants without scoliosis, who had declining function with restrictive disease, suggesting that the pulmonary dysfunction of OI is attributable to a primary defect in lung related to structurally abnormal collagen. The studies are important because pulmonary issues are the most prevalent cause of morbidity and mortality in OI, and affected individuals should now seek anticipatory evaluation and treatment.
Our randomized controlled trial of bisphosphonate in children with types III and IV OI was the first randomized trial in the United States and one of four worldwide. Our trial examined both direct skeletal and secondary gains reported in uncontrolled trials. For skeletal outcomes, we found increased BMD z-scores and improved vertebral area and compressions. We noted that vertebral BMD improvement tapered off after two years treatment. Our treatment group did not experience fewer long-bone fractures, coinciding with the lack of improvement or equivocal improvement in fractures in other controlled trials. The BEMB controlled trial did not support the secondary gains claimed in observational trials, including improvement in ambulation level, lower-extremity strength, or alleviation of pain, suggesting these were placebo effects in observational trials. Our current recommendation is treatment for 2–3 years, with subsequent follow-up of bone status. We are now engaged in a dose-comparison trial, comparing the dose from our first trial with a lower dose, achieved by increasing the cycle interval at the same dose/kg/cycle. Given the decade-long half-life and side effects of bisphosphonate on normal as well as dysplastic bone, including osteonecrosis of the jaw (ONJ), bone healing, bone modeling, and decline in the quality of the bone material, it is important to determine the lowest cumulative dose that will provide vertebral benefits. Preliminary analysis indicates that OI children obtain benefits from lower pamidronate doses that are comparable to the benefits from higher doses.
OI Mutation Consortium
The BEMB assembled and leads an international consortium of connective tissue laboratories for the compilation and analysis of a database of mutations in type I collagen that cause OI. The Consortium Database assembled double the previously available number of collagen mutations, and when the first analysis of the database was published in 2007, it listed over 830 mutations, including 682 glycine substitutions and 150 splice-site defects. Genotype-phenotype modeling revealed distinct functions for each alpha chain of type I collagen, including the occurrence of exclusively lethal mutations in the Major Ligand Binding Regions (MLBR) of the α1(I) chain on the collagen monomer and the overlapping of the regularly spaced clusters of lethal mutations along the α2(I) chain with the proteoglycan binding sites on the collagen fibril. The modeling for α2(I) supports the Regional Model for mutation that was first proposed by the BEMB over 15 years ago and now correctly predicts 86% of clinical outcomes. The Consortium Database has provided the basis for our collaborators James San Antonio and Joseph Orgel to model functional domains in terms of the cell and matrix interactions of the collagen fibril. The Consortium Database now contains over 1,570 mutations from nine international laboratories. An upcoming analysis of this database will also examine the effects of interchain salt bridges and re-nucleation residues C-terminal to the substituted glycine.
Additional Funding
- NICHD Director's Award
Publications
- Barnes AM, Duncan G, Weis MA, Paton W, Cabral WA, Mertz EL, Makareeva E, Gambello MJ, Lacbawan FL, Leikin S, Fertala A, Eyre DR, Bale SJ, Marini JC. Kuskokwim syndrome, a recessive congential contracture disorder, extends the phenotype of FKBP10 mutations. Hum Mutat 2013;34:1279-1288.
- Thiele F, Cohrs CM, Flor A, Lisse TS, Przemeck GHK, Horsch M, Schrewe A, Gialus-Durner V, Ivandic B, Katus H, Wurst W, Reisenberg C, Chaney H, Fuchs H, Hans W, Beckers J, Marini JC, Hrabe de Angelis M. Cardiopulmonary dysfunction in the osteogenesis imperfect mouse model aga2 and human patients are caused by bone-independent mechanisms. Hum Mol Genet 2012;21(16):3535-3545.
- Lindahl K, Barnes AM, Fratzl-Zelman N, Whyte MP, Hefferan TE, Makareeva E, Brusel M, Yaszemski MJ, Rubin CJ, Kindmark A, Roschger P, Klaushofer K, McAlister WH, Mumm S, Leikin S, Kessler E, Boskey AL, Ljunggren O, Marini JC. COL1 C-propeptide cleavage site mutations cause high bone mass osteogenesis imperfecta. Hum Mat 2011;32:598-609.
- Farber CR, Reich A, Barnes AM, Becerra P, Rauch F, Cabral WA, Bae A, Quinlan A, Glorieux FH, Clemens TL, Marini JC. A novel IFITM5 mutation in severe atypical osteogenesis imperfect type VI impairs osteoblast production of PEDF. J Bone Miner Res 2014;29:1402-1411.
- Cabral WA, Perdivara I, Weis MA, Terajima M, Blissett AR, Chang W, Makareeva EN, Mertz E, Leikin S, Tomer KB, Eyre DR, Yamauchi M, Marini JC. Abnormal type I collagen post-translational modification and crosslinking in a cyclophilin B KO mouse model of recessive osteogenesis imperfecta. PLoS Genetics 2014;10(6):e1004465.
Collaborators
- Adele Boskey, PhD, Weill Medical College of Cornell University, New York City
- David Eyre, PhD, University of Washington, Seattle, WA
- Steven Goldstein, PhD, University of Michigan, Ann Arbor, MI
- Martin Hrabe de Angelis, PhD, Institute of Experimental Genetics, Helmholtz Zentrum München, Munich, Germany
- Kenneth Kozloff, PhD, University of Michigan, Ann Arbor, MI
- Sergey Leikin, PhD, Section on Physical Biochemistry, NICHD, Bethesda, MD
- Katarina Lindahl, MD, Uppsala University, Uppsala, Sweden
- Joseph Orgel, PhD, Illinois Institute of Technology, Chicago, IL
- Philip Osdoby, PhD, Washington University, St. Louis, MO
- Scott Paul, MD, Rehabilitation Medicine, NIH Clinical Center, Bethesda, MD
- Timothy Rebbeck, PhD, University of Pennsylvania, Philadelphia, PA
- Charles N. Rotimi, PhD, NIH Center for Research on Genomics and Global Health, NHGRI, Bethesda, MD
- James San Antonio, PhD, Jefferson University, Philadelphia, PA
- Sarah Tishkoff, PhD, University of Pennsylvania, Philadelphia, PA
- Joseph Wallace, PhD, University of Michigan, Ann Arbor, MI
- Bernd Wollnik, MD, Zentrum für Molekulare Medizin Köln, Uniklinik Köln, Cologne, Germany
- The OI Mutation Consortium, NICHD, Bethesda, MD
Contact
For more information, email marinij@mail.nih.gov.