You are here: Home > Laboratory of Genomic Integrity
Studies on DNA Replication, Repair, and Mutagenesis in Eukaryotic and Prokaryotic Cells
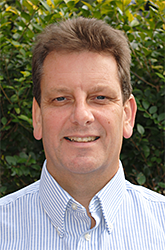
- Roger Woodgate, PhD, Head, Laboratory of Genomic Integrity
- Ekaterina Chumakov, PhD, Staff Scientist
- Alexandra Vaisman, PhD, Interdisciplinary Scientist
- John McDonald, PhD, Biologist
- Mary McLenigan, BS, Chemist
- Sender Aspelund, PhD, Postdoctoral Intramural Research Training Award Fellow
- Ewa Grabowska, PhD, Postdoctoral Intramural Research Training Award Fellow
- Erin Walsh, PhD, Postdoctoral Intramural Research Training Award Fellow
- Donald Huston, BS, Technical Intramural Research Training Award Trainee
- David Wilson, BS, Postbaccalaureate Intramural Research Training Award Fellow
- Hallie Whalen, Student Fellow
Under optimal conditions, the fidelity of DNA replication is extremely high. Indeed, it is estimated that, on average, only one error occurs for every 10 billion bases replicated. However, given that living organisms are continually subjected to a variety of endogenous and exogenous DNA–damaging agents, optimal conditions rarely prevail in vivo. While all organisms have evolved elaborate repair pathways to deal with such damage, the pathways rarely operate with 100% efficiency. Thus, the persisting DNA lesions are replicated, but with much lower fidelity than in undamaged DNA. Our aim is to understand the molecular mechanisms by which mutations are introduced into damaged DNA. The process, commonly referred to as translesion DNA synthesis (TLS), is facilitated by one or more members of the Y-family of DNA polymerases, which are conserved from bacteria to humans. Based on phylogenetic relationships, Y-family polymerases may be broadly classified into five subfamilies: DinB-like (polIV/pol kappa–like) proteins are ubiquitous and found in all domains of life; in contrast, the Rev1-like, Rad30A (pol eta)–like, and Rad30B (pol iota)–like polymerases are found only in eukaryotes, and the UmuC (polV)–like polymerases only in prokaryotes. We continue to investigate TLS in all three domains of life: bacteria, archaea, and eukaryotes.
Prokaryotic DNA repair and mutagenesis
Most damage-induced mutagenesis in Escherichia coli depends on the UmuD′C protein complex, which contains DNA polymerase V (pol V) (1). Pol V has intrinsically weak catalytic activity, but is dramatically stimulated by interactions with ATP and RecA. In a collaborative study with Myron Goodman, we recently established three features of the roles of ATP and RecA in the activation of pol V: i) RecA–activated DNA polymerase V (pol V Mut), is a DNA–dependent ATPase; ii) bound ATP is required for DNA synthesis; iii) pol V Mut function is regulated by ATP, with ATP required to bind to primer-template DNA and ATP hydrolysis triggering dissociation from the DNA. Pol V Mut formed with an ATPase–deficient RecA E38K/K72R mutant hydrolyzes ATP rapidly, thereby establishing the DNA–dependent ATPase as an intrinsic property of pol V Mut distinct from the ATP hydrolytic activity of RecA when bound to single-stranded (ss)DNA as a nucleoprotein filament (RecA*) (2). To our knowledge, no similar ATPase activity or autoregulatory mechanism has been found previously for a DNA polymerase.
Low-fidelity DNA pol V is best characterized for its ability to perform TLS. However, in recA730 lexA(Def) strains, the enzyme is expressed under optimal conditions, allowing it to compete with the cell’s replicase for access to undamaged chromosomal DNA, which leads to a substantial increase in spontaneous mutagenesis. We recently showed that a Y11A substitution in the “steric gate” residue of UmuC reduces both base and sugar selectivity of pol V, but instead of generating an increased number of spontaneous mutations, strains expressing umuC_Y11A are poorly mutable in vivo. The phenotype is attributed to efficient RNase HII–initiated repair of the misincorporated ribonucleotides, which concomitantly removes adjacent misincorporated deoxyribonucleotides. To investigate the mechanisms underlying ribonucleotide excision repair (RER), we utilized the ability of the pol V steric-gate mutant to promote incorporation of large numbers of errant ribonucleotides into the E. coli genome. In the past year, we demonstrated that RER is normally facilitated by DNA polymerase I (pol I) via classical “nick translation” (3). In vitro, pol I displaces 1–3 nucleotides of the RNA/DNA hybrid and, through its 5′-3′ (exo/endo) nuclease activity, releases ribo- and deoxyribonucleotides from DNA. In vivo, umuC_Y11A–dependent mutagenesis changes significantly in polymerase-deficient or proofreading-deficient polA strains, indicating a pivotal role for pol I in RER. However, there is also considerable redundancy in the RER pathway in E. coli. Pol I’s strand displacement and FLAP–exo/endonuclease activities can be facilitated by alternate enzymes, while the DNA polymerization step can be assumed by high-fidelity pol III. Based on these observations, we concluded that RNase HII and pol I normally act to minimize the genomic instability that is generated through errant ribonucleotide incorporation, but that the “nick-translation” activities encoded by the single pol I polypeptide can be undertaken by a variety of back-up enzymes.
The umuC_Y11A mutant not only allows pol V to readily misincorporate ribonucleotides as frequently as deoxynucleotides, it also leaves its poor base-substitution fidelity essentially unchanged. However, the mutability of cells expressing the umuC_Y11A mutant is very low as a consequence of efficient repair mechanisms that are triggered by the misincorporated ribonucleoside monophosphates (rNMPs). Comparison of the mutation frequency between strains expressing wild-type and mutant pol V therefore allowed us to identify pathways specifically directed at RER. As noted above, we recently demonstrated that rNMPs incorporated by umuC_Y11A are efficiently removed from DNA in a repair pathway initiated by RNase HII. Indeed, in the absence of RNase HII, umuC_Y11A–dependent mutagenesis increased from about 7% of that observed with wild-type pol V to roughly 40% of wild-type levels. However, based on the biochemical properties of umuC_Y11A, we expected the mutant to be as mutable as wild-type pol V, if not more so, suggesting the existence of additional DNA repair pathways that not only remove misincorporated ribonucleotides, but misincorporated deoxyribonucleotides. In the past year, we discovered that mismatch repair and base excision repair play only minimal back-up roles in RER in vivo. In contrast, in the absence of functional RNase HII, umuC_Y11A–dependent mutagenesis increased significantly in ∆uvrA, uvrB5, and ∆uvrC strains, suggesting that rNMPs misincorporated into DNA are actively repaired by nucleotide excision repair (NER) in vivo (4). Participation of NER in RER was confirmed by reconstituting ribonucleotide-dependent NER in vitro. We showed that UvrABC nuclease–catalyzed incisions are readily made on DNA templates containing one, two, or five rNMPs and that the reactions are stimulated by the presence of mispaired bases. Similar to NER of DNA lesions, excision of rNMPs proceeds through dual incisions made at the eighth phosphodiester bond 5′ and fourth–fifth phosphodiester bonds 3′ of the ribonucleotide. Ribonucleotides misinserted into DNA can therefore be added to the broad list of helix-distorting modifications that are substrates for NER.
Eukaryotic DNA repair and mutagenesis
Our studies on the human TLS polymerases focused on the ability of a Y39A “steric gate” mutant of human DNA polymerase iota to incorporate ribonucleotides into DNA (5). This is of considerable interest, given that the enzyme has been implicated in translesion DNA synthesis of both oxidative and UV–induced lesions. We discovered that human pol iota incorporates and extends nucleoside triphosphates (NTPs) opposite both damaged and undamaged template bases in a template-specific manner. The Y39A pol iota mutant was considerably more active in the presence of Mn2+ compared with Mg2+ and exhibited a marked increase in NTP incorporation and extension; surprisingly, it also exhibited increased dNTP base selectivity. Our results indicate that a single residue in pol iota is able to discriminate between NTPs and deoxyribonucleotide triphosphates (dNTPs) during DNA synthesis. Given that wild-type pol iota incorporates NTPs in a template-specific manner, we believe that certain DNA sequences may be “at-risk” for elevated mutagenesis during pol iota–dependent TLS. Molecular modeling indicated that the constricted active site of wild-type pol iota becomes more spacious in the Y39A variant. Therefore, the Y39A substitution not only permits incorporation of ribonucleotides, but also causes the enzyme to favor faithful Watson-Crick base pairing over mutagenic configurations.
Additional Funding
- NIH Director’s Challenge Award
Publications
- Goodman MF, Woodgate R. Translesion DNA polymerases. Cold Spring Harb Perspect Biol 2013;5:a010363.
- Erdem AL, Jaszczur M, Bertram JG, Woodgate R, Cox MM, Goodman MF. DNA polymerase V activity is autoregulated by a novel intrinsic DNA-dependent ATPase. eLife 2014;3:e02384.
- Vaisman A, McDonald JP, Noll S, Huston D, Loeb G, Goodman MF, Woodgate R. Investigating the mechanisms of ribonucleotide excision repair in Escherichia coli. Mutat Res Fundam Mol Mech Mutagen 2014;761:21-33.
- Vaisman A, McDonald JP Huston D, Kuban W, Liu L, Van Houten B, Woodgate R. Removal of misincorporated ribonucleotides from prokaryotic genomes: an unexpected role for nucleotide excision repair. PLoS Genet 2013;9:e1003878.
- Donigan KA, McLenigan MP, Yang W, Goodman MF, Woodgate R. The steric gate of DNA polymerase iota regulates ribonucleotide incorporation and deoxyribonucleotide fidelity. J Biol Chem 2014;289:9136-9145.
Collaborators
- Michael Cox, PhD, University of Wisconsin, Madison, WI
- Myron F. Goodman, PhD, University of Southern California, Los Angeles, CA
- Bennett Van Houten, PhD, University of Pittsburgh, Pittsburgh, PA
- Wei Yang, PhD, Laboratory of Molecular Biology, NIDDK, Bethesda, MD
Contact
For more information, email woodgate@mail.nih.gov or visit www.nichd.nih.gov/research/atNICHD/Investigators/woodgate.