You are here: Home > Unit on Human Copper Metabolism
Viral Gene Therapy for Neurometabolic Disorders
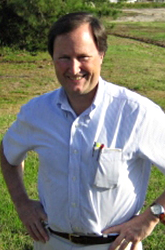
- Stephen G. Kaler, MD, Head, Unit on Human Copper Metabolism
- Eun-Young Choi, PhD, Postdoctoral Fellow
- Anthony Donsante, PhD, Postdoctoral Fellow*
- *Current junior faculty appt, Emory University, former Postdoctoral Fellow
- Marie-Reine Haddad, PhD, Postdoctoral Fellow
- Ling Yi, PhD, Postdoctoral Fellow
- Julia D. Hicks, BS, Postbaccalaureate Fellow**
- **Current Medical student, former Postbaccalaureate Fellow
- Joshua A. Lee, BA, Postbaccalaureate Fellow
The Unit on Human Copper Metabolism strives to dissect and understand mechanisms of metabolic disease and to use the knowledge gained to develop treatments, including gene therapy. Patients and families affected by inborn errors of metabolism provide the impetus for scientific inquiry. In addition to molecular genetics, the laboratory employs model organisms (mouse, yeast), cellular, biochemical, and biophysical approaches and conducts clinical trials. The Laboratory has two main current projects: (i) viral gene therapy in mouse models of human monogenic neurometabolic disease, and (ii) the mechanisms responsible for normal and abnormal intracellular trafficking of the closely related copper-transporting P-type ATPases ATP7A and ATP7B.
Adeno-associated viral (AAV) gene therapy for neurometabolic diseases
Brain-directed AAV5 gene therapy in mo-br male mice, a mouse model of Menkes disease, resulted in rescue from early lethality in approximately 30% of affected males, via efficient transduction of choroid plexus (CP) epithelia, which resulted in elevation of brain copper concentrations. CP tissues are highly vascularized structures that project into the ventricles of the brain. Besides creating the blood-CSF barrier, the polarized epithelia of the CP produce CSF by transporting water and ions into the ventricles from the blood and secreting a large number of proteins. We believe that another category of neurometabolic disease, lysosomal storage diseases, could benefit from a CP–targeted approach, given that rAAV transduction results in sustained episomal transgene expression and that CP epithelia have a slow turnover rate. CSF flow extends throughout the ventricular system to the subarachnoid space, from which molecules ultimately reach the entire brain.
Based on the above observation that concentrated transduction of CP epithelia enabled rescue of the mo-br mouse, we are developing AAV vectors selectively evolved to target the specialized cells of the CP by phage panning. The technique has been used to identify viral capsid peptide motifs that allow superior vector homing to specific cells and tissues. We identified three motifs that demonstrated enhanced binding to wild-type mouse CP epithelia after serial rounds of phage panning. We are currently cloning the motifs into the rAAV2 capsid between amino acid residues 587N and 588R. Insertion at this site destroys the affinity of AAV2 for heparin sulfate receptors and has been used previously to redirect rAAVs to specific cell types. We will use a GFP reporter cassette containing a CP-specific promoter (transthyretin) for formal testing of these novel vectors in mice.
Such choroid plexus–specific vectors could be especially relevant to gene therapy of lysosomal storage diseases (LSDs) that impact the CNS. Intrathecal delivery of recombinant lysosomal enzymes (injecting enzyme into the cerebrospinal fluid during a spinal tap) has been successful in ameliorating LSDs in some animal studies and in human clinical trials. However, a major drawback to this approach is the need for repeated (e.g., monthly) intrathecal injections due to short half-lives of recombinant enzymes. An alternative strategy is to remodel CP epithelial cells with an AAV vector containing the cDNA for the enzyme of interest. Given that CP epithelia have an extremely slow turnover rate, this approach could generate a permanent source of enzyme production for secretion into the CSF and penetration into cerebral and cerebellar structures. Our 2012 NIH Bench-to-Bedside Award, entitled "Choroid plexus-directed gene therapy: Toward novel clinical management of lysosomal storage disease," supports evaluation of this hypothesis.
In collaboration with John Wolfe, we are evaluating the efficacy of two AAV serotypes known to transduce CP epithelia (AAV4, AAV5), as well as the novel CP–permissive AAV capsid variants mentioned above, in animal models of alpha-mannosidosis, a prototypical lysosomal storage disease. We will use both mouse and guinea pig models of alpha-mannosidosis to evaluate choroid plexus transduction by the vectors as well as post-treatment alpha-mannosidase concentration and distribution in brain. Studies in the mouse model (obtained by NICHD through a Material-CRADA with the University of Kiel, Germany) will require less virus and be easier to breed. The guinea pig model (housed at the University of Pennsylvania) features a partially gyrencephalic brain more similar to the human brain; thus the study of these two models will be complementary. In a related study, we are collaborating with Patricia Dickson to compare the efficiency of CP–mediated lysosomal enzyme production with intrathecal enzyme replacement in animal models of mucopolysaccharidoses. The latter project was selected for funding (of both laboratories) by the National MPS Society.
Disease mechanisms that underlie ATP7A–related distal motor neuron degeneration
The P-type ATPase ATP7A regulates cellular copper homeostasis by its activity at the trans-Golgi network (TGN) and plasma membrane (PM), with location normally governed by intracellular copper concentration. In addition to causing Menkes disease, defects in ATP7A may lead to the disease variants occipital horn syndrome and to ATP7A–related distal motor neuropathy, a newly discovered adult-onset condition for which the precise pathophysiology has been obscure. We characterized the two ATP7A motor neuropathy mutations (T994I, P1386S) and identified molecular mechanisms for abnormal intracellular trafficking. In the patients' fibroblasts, total internal reflection fluorescence (TIRF) microscopy indicated a shift in steady-state equilibrium of ATP7AT994I and ATP7AP1386S, with excess plasma membrane accumulation. Transfection of 293T cells and NSC-34 motor neurons with the mutant alleles tagged with Venus fluorescent protein also showed enhanced plasma membrane localization and delayed endocytic retrieval of the mutant alleles to the trans-Golgi.
Immunoprecipitation assays revealed an abnormal interaction between ATP7AT994I and p97/VCP (valosin-containing protein), a protein that normally associates with the endocytic trafficking proteins clathrin and early endosomal autoantigen 1 (EEA1) and which is mutated in two autosomal dominant forms of motor neuron disease: amyotrophic lateral sclerosis and inclusion body myopathy with early-onset Paget disease and fronto-temporal dementia. Small-interfering RNA (siRNA) knockdown of p97/VCP corrected ATP7AT994I mislocalization. VCP did not interact significantly with ATP7AP1386S, the other mutant allele associated with the motor neuropathy phenotype. However, flow cytometry documented that non-permeabilized ATP7AP1386S fibroblasts bound to a carboxyl-terminal ATP7A antibody, a finding consistent with partially destabilized insertion of the eighth transmembrane helix and relocation of the di-leucine endocytic retrieval signal from the cytosolic to the extracellular face of the plasma membrane. The findings illuminated mechanisms underlying ATP7A–related distal motor neuropathy, established a common link between genetically distinct forms of motor neuron disease, clarified the normal process of ATP7A endocytosis, and highlighted the possible functional role of ATP7A in the peripheral nervous system. We recently extended our studies on this topic to elucidate the specific binding regions between p97/VCP and ATP7AT994I. We are also investigating the normal trafficking of ATP7A and of ATP7B, a closely related copper ATPase associated with Wilson disease. Interaction with clathrin-coated vesicles and adaptor protein complexes, as well as post-translational modification by palmitoylation are among topics under active investigation. Through these studies, we hope to resolve unanswered questions concerning the molecular mechanisms of altered copper-ATPase intracellular trafficking.
Clinical protocols
- 1. Principal Investigator, 90-CH-0149: Early copper histidine treatment in Menkes disease: relationship of molecular defects to neurodevelopmental outcomes.
- Associate Investigator, 02-CH-0023: Studies of pediatric patients with metabolic or other genetic disorders.
- Principal Investigator, 09-CH-0059: Molecular bases of response to copper treatment in Menkes disease, related phenotypes, and unexplained copper deficiency.
Patent filed
Patent 4239-81164-01, Identification of subjects likely to benefit from copper treatment. International Filing Date: 06 October 2008
Additional Funding
- National Mucopolysaccharidosis Society
- NIH Bench-to-Bedside Program
Publications
- Kaler SG. ATP7A-related copper transport diseases—emerging concepts and future trends. Nat Rev Neurol 2011;7:15-29.
- Donsante A, Yi L, Zerfas P, Brinster L, Sullivan P, Goldstein DS, Prohaska J, Centeno JA, Kaler SG. ATP7A gene addition to the choroid plexus results in long-term rescue of the lethal copper transport defect in a Menkes disease mouse model. Mol Ther 2011;19:2114-2123.
- Yi L, Donsante A, Kennerson ML, Mercer JFB, Garbern JY, Kaler SG. Altered intracellular localization and valosin-containing protein (p97 VCP) interaction underlie ATP7A-related distal motor neuropathy. Hum Mol Genet 2012;21:1794-1807.
- Huppke P, Brendel C, Kahlscheuer V, Korenke GC, Marquardt I, Freisinger P, Christodoulou J, Pitelet G, Wilson C, Gruber-Sedlmayr U, Ullmann R, Haas S, Elpeleg O, Nürnberg G, Nürnberg P, Dad S, Møller LB, Kaler SG, Gärtner J. Mutation in SLC33A1, a highly conserved acetyl-transferase, causes a lethal autosomal recessive syndrome of congenital cataracts, hearing loss, and neurodegeneration. Am J Hum Genet 2012;90:61-68.
- Donsante A, Sullivan P, Goldstein DS, Brinster LR, Kaler SG. Systemic L-threo dihydroxyphenylserine corrects neurochemical abnormalities in a mouse model of Menkes disease. Ann Neurol 2013;in press.
Collaborators
- Lauren Brinster, VMD, Division of Veterinary Resources, Office of Research Services, NIH, Bethesda, MD
- Jose Centeno, PhD, Walter Reed Army Medical Center, Silver Spring, MD
- Christopher Chang, PhD, University of California-Berkeley, Berkeley, CA
- John Chiorini, PhD, Molecular Physiology and Therapeutics Branch, NIDCR, Bethesda, MD
- John Christodoulou, MD, University of Sydney, Sydney, Australia
- Laurence Colleaux, PhD, INSERM, Paris, France
- Soma Das, PhD, University of Chicago, Chicago, IL
- Patricia Dickson, MD, Harbor-UCLA Medical Center, Los Angeles, California
- Tohru Fukai, MD, PhD, University of Illinois at Chicago, Chicago, IL
- James Y. Garbern, MD, PhD, Wayne State University School of Medicine, Detroit, MI
- Jutta Gartner, MD, Georg August Universität, Göttingen, Germany
- David S. Goldstein, MD, PhD, Clinical Neurosciences Program, NINDS, Bethesda, MD
- George Grimes, RPh, Pharmaceutical Development Service, Clinical Center, NIH, Bethesda, MD
- Courtney Holmes, CMT, Clinical Neurosciences Program, NINDS, Bethesda, MD
- Peter Huppke, MD, Georg August Universität, Göttingen, Germany
- Marina L. Kennerson, PhD, University of Sydney, Sydney, Australia
- Robert Kotin, PhD, Laboratory of Molecular Virology and Gene Therapy, NHLBI, Bethesda, MD
- Julian Mercer, PhD, Deakin University, Melbourne, Australia
- Nicholas Patronas, MD, Diagnostic Radiology Department, Clinical Center, NIH, Bethesda, MD
- Joseph Prohaska, PhD, University of Minnesota, Duluth, MN
- Yulia Pushkar, PhD, Purdue University, West Lafayette, IN
- Paul Saftig, PhD, Christian-Albrechts-Universität, Kiel, Germany
- Judith Starling, RPh, Pharmaceutical Development Service, Clinical Center, NIH, Bethesda, MD
- Peter Steinbach, PhD, Center for Molecular Modeling, CIT, NIH, Bethesda, MD
- Vinzenz Unger, PhD, Northwestern University, Evanston, IL
- John Wolfe, VMD, PhD, University of Pennsylvania, Philadelphia, PA
- Wei Zheng, PhD, Purdue University, West Lafayette, IN