You are here: Home > Section on Intracellular Protein Trafficking
Protein Sorting in the Endosomal-Lysosomal System
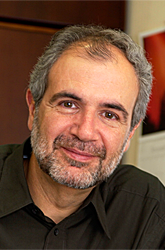
- Juan S. Bonifacino, PhD, Head, Section on Intracellular Protein Trafficking
- Patricia V. Burgos, PhD, Postdoctoral Fellow
- Rittik Chaudhuri, BS, Postbaccalaureate Fellow
- Luis L. P. DaSilva, PhD, Postdoctoral Fellow
- Shuhei Ishikura, PhD, Postdoctoral Fellow
- Javier G. Magadán, PhD, Postdoctoral Fellow
- Gonzalo A. Mardones, PhD, Postdoctoral Fellow
- Rafael Mattera, PhD, Staff Scientist
- Yogikala Prabhu, PhD, Postdoctoral Fellow
- F. Javier Pérez-Victoria, PhD, Postdoctoral Fellow
- Raúl Rojas, PhD, Postdoctoral Fellow
- Christina A. Schindler, PhD, Postdoctoral Fellow
- Krishnakant Soni, PhD, Postdoctoral Fellow
- Kathryn E. Tifft, PhD, Postdoctoral Fellow
- Xiaolin Zhu, RN, Technician
We investigate the molecular mechanisms by which transmembrane proteins are sorted to intracellular compartments such as endosomes, lysosomes, and a group of cell type–specific organelles known as lysosome-related organelles (e.g., melanosomes and platelet dense bodies). Sorting to these compartments is mediated by recognition of signals present in the cytosolic domains of the transmembrane proteins by adaptor proteins that are components of membrane coats. Among these adaptor proteins are the heterotetrameric AP-1, AP-2, AP-3, and AP-4 complexes, the monomeric GGA1, GGA2, and GGA3 proteins (GGAs) (see image below), and the heteropentameric retromer complex. Proper sorting also requires the function of other components of the trafficking machinery that mediate vesicle tethering and fusion, such as the heterotetrameric GARP complex. Current work in our laboratory aims to elucidate the structure, regulation, and physiological roles of coat proteins and vesicle tethering factors and investigating pathological states involving dysfunction of these proteins (e.g., the Hermansky-Pudlak syndrome, Alzheimer's Disease, HIV pathogenesis).
Mechanisms of CD4 downregulation by the Nef and Vpu proteins of HIV-1
The Nef protein of HIV-1 is an important determinant of pathogenicity, as demonstrated by the finding that some long-term non-progressors carry an HIV-1 strain with inactivating mutations of the Nef gene. Nef has many effects in infected cells, the best characterized of which is the downregulation of CD4. Previous work in our laboratory demonstrated that the downregulation of CD4 by Nef requires clathrin and the heterotetrameric, clathrin-associated adaptor protein-2 (AP-2) complex in host cells. In addition, we demonstrated that this requirement stemmed from a direct interaction of Nef with AP-2 involving a dileucine and a diacidic motif in Nef and the alpha and sigma2 subunits of AP-2. Through mutational analyses, we mapped the binding site for both Nef motifs on alpha and sigma2 (Chaudhuri et al., 2009). Given that clathrin and AP-2 mediate endocytosis from the plasma membrane, these findings indicated that Nef downregulates CD4 by accelerating its internalization from the surface of host cells. This past year, we discovered a new function of Nef: the targeting of internalized CD4 to the multivesicular body (MVB) pathway for eventual degradation in lysosomes (daSilva et al., 2009). We found that this targeting occurred independently of ubiquitination but required the ESCRT complexes that deliver cargo to intraluminal vesicles of MVBs. At later stages of infection, the HIV-1 genome directs expression of a second protein, Vpu, that also downregulates CD4. Vpu inserts into the membrane of the endoplasmic reticulum (ER) and targets newly synthesized CD4 for degradation in cytosolic proteasomes. The mechanism of this targeting was thought to be distinct from the canonical ER-associated degradation (ERAD) pathway. However, in collaboration with Klaus Strebel (NIAID) and Yihong Ye (NIDDK), we found that CD4 downregulation by Vpu involves at least part of the ERAD pathway. In particular, the p97-Npl4-Ufd1 ERAD dislocase is required for Vpu-induced CD4 targeting for proteasomal degradation. We also found that, in addition to ERAD targeting, Vpu induces CD4 retention in the ER. This retention is largely dependent on transmembrane domain interactions.
Cleft palate caused by a defect in AP-2
Mutant organisms have previously aided in investigations of the physiological roles of AP complexes. For example, in collaboration with William Gahl (NHGRI), we had shown that mutations in one of the subunits of the AP-3 complex in humans cause the pigmentation and bleeding disorder Hermansky-Pudlak syndrome type 2. To investigate the role of AP-2, we had previously ablated the gene encoding the mu2 subunit in mice. This approach yielded little information because defective expression of mu2 resulted in early embryonic lethality. In collaboration with Eric Everett, we characterized a mutant mouse deficient in expression of the beta2 subunit of AP-2. Unlike the mu2 mutant mouse, the beta2 mutant mouse survives until birth but dies shortly thereafter. The viability of beta2 mutant embryos is attributable to substitution of the beta1 subunit of AP-1 for beta2 in the AP-2 complex. Most interestingly, the newborn beta2 mutant mouse exhibits a single visible developmental defect: cleft palate. Thus, endocytosis must be critical for the development of the palate, probably by promoting the internalization of a key signaling receptor. Our finding identifies a possible cause for cleft palate, which is one of the most frequently occurring congenital deformities in humans.
Role of the GARP complex in transport from endosomes to the Golgi complex
Endosomal transport carriers formed by the action of retromer must dock at and fuse with the trans-Golgi network (TGN) in order to deliver their cargo. We previously showed that GARP, a multi-protein complex originally described in yeast, plays such a role in mammalian cells. Interference with GARP blocks the delivery of cargos such as mannose 6-phosphate receptors and Shiga toxin from endosomes to the TGN, indicating that GARP has a general role in retrograde transport. A mutation in one of the GARP subunits, Vps54, was recently identified in the Wobbler mouse mutant, an animal model of amyotrophic lateral sclerosis. We found that the Wobbler mutation does not prevent the function of GARP in retrograde transport, suggesting that the disease is likely attributable to a subtle defect in transport or to some other function of GARP. Further investigation of the molecular mechanism of GARP function showed that this complex plays two distinct roles in retrograde transport: 1) tethering of vesicular transport intermediates to the TGN; and 2) interaction with the SNARE proteins Syntaxin 6, Syntaxin 16, and Vamp4 at the TGN in a way that promotes their assembly into SNARE complexes. GARP thus orchestrates the tethering and fusion of retrograde transport intermediates by participating in two consecutive, independent steps (Pérez-Victoria et al., 2009).
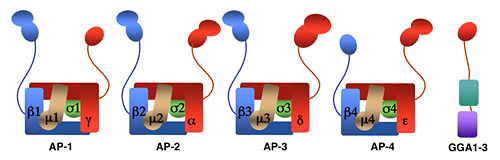
Figure 1. Structure of AP complexes and GGAs.
Sorting of the amyloid precursor protein regulated by AP-4
The AP-4 complex is the most-recently discovered and least understood of the family of AP complexes. In recent work, we have found that AP-4 interacts with the cytosolic tail of the Alzheimer's disease (AD) amyloid precursor protein (APP). Disruption of the AP-4-APP interaction shifts the distribution of APP from endosomes to the TGN and enhances gamma-secretase–catalyzed processing of APP to the pathogenic amyloid-beta peptide. These findings establish AP-4 as a novel regulator of APP processing and trafficking and as a potential risk factor for AD.
Regulation of the retromer complex by the Rab7 GTPase
The retromer complex is a sorting device that mediates retrograde transport from endosomes to the TGN. This function is essential for many important physiological processes in higher eukaryotes, including lysosomal enzyme sorting, processing of APP, and formation of morphogen gradients during development. The retromer consists of a membrane-binding subcomplex made up of two sorting nexin (SNX) subunits and a cargo-recognition subcomplex composed of Vps26, Vps29, and Vps35. In previous studies, we discovered a role for the retromer complex in the retrieval of mannose 6-phosphate receptors from endosomes to the TGN and showed that this retrieval is essential for acid hydrolase sorting to lysosomes. In collaboration with James Hurley, we also solved the crystal structure of the Vps26-Vps29-Vps35 complex. Over the past year, we found that the recruitment of the Vps26-Vps29-Vps35 subcomplex to membranes involves, in addition to the SNX subcomplex, the small GTP-binding protein Rab7 (Rojas et al., 2008). Perturbation of either the SNX subcomplex or Rab7 results in dissociation of the Vps26-Vps29-Vps35 subcomplex from membranes. In turn, this leads to missorting of acid hydrolases and consequent accumulation of undegraded materials in lysosomes, a phenotype characteristic of lysosomal storage disorders.
Additional Funding
- Intramural AIDS Targeted Antiviral Program (IATAP)
Publications
- Chaudhuri R, Mattera R, Lindwasser OW, Robinson MS, Bonifacino JS. A basic patch on alpha-adaptin is required for binding of human immunodeficiency virus type 1 Nef and cooperative assembly of a CD4-Nef-AP-2 complex. J Virol 2009 83:2518-2530.
- daSilva LL, Sougrat R, Burgos PV, Janvier K, Mattera R, Bonifacino JS. Human immunodeficiency virus type 1 Nef protein targets CD4 to the multivesicular body pathway. J Virol 2009 83:6578-6590.
- Mattera R, Bonifacino JS. Ubiquitin binding and conjugation regulate the recruitment of Rabex-5 to early endosomes. EMBO J 2008 27:2484-2494.
- Pérez-Victoria FJ, Bonifacino JS. Dual roles of the mammalian GARP complex in tethering and SNARE complex assembly at the trans-Golgi network. Mol Cell Biol 2009 29:5251-5263.
- Rojas R, Van Vlijmen T, Mardones GA, Prabhu Y, Rojas AL, Mohammed S, Heck AJR, Raposo G, van der Sluijs P, Bonifacino JS. Regulation of retromer recruitment to endosomes by sequential action of Rab5 and Rab7. J Cell Biol 2008 183:513-526.
Collaborators
- Eric T. Everett, PhD, University of North Carolina, Chapel Hill, NC
- James H. Hurley, PhD, Laboratory of Molecular Biology, NIDDK, Bethesda, MD
- Catherine L. Jackson, PhD, CNRS, Gif sur Yvette, France
- Caroline C. Philpott, MD, Liver Diseases Branch, NIDDK, Bethesda, MD
- Graça Raposo, PhD, Curie Institute, Paris, France
- Margaret S. Robinson, PhD, University of Cambridge, Cambridge, UK
- Klaus Strebel, PhD, Laboratory of Molecular Microbiology, NIAID, Bethesda, MD
- Peter Van der Sluijs, PhD, Utrecht University School of Medicine, Utrecht, The Netherlands
- Yihong Ye, PhD, Laboratory of Molecular Biology, NIDDK, Bethesda, MD
Contact
For further information, contact juan@helix.nih.gov or bonifacinoj@mail.nih.gov, or visit cbmp.nichd.nih.gov/sipt.