You are here: Home > Program on Pediatric Imaging and Tissue Sciences
Quantitative Biophotonics for Tissue Characterization and Function
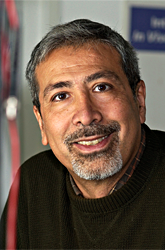
- Amir H. Gandjbakhche, PhD, Head, Section on Analytical and Functional Biophotonics
- Victor Chernomordik, PhD, Staff Scientist
- Moinuddin Hassan, PhD, Research Fellow
- Jason Riley, PhD, Postdoctoral Fellow
- Jana Kainerstorfer, MS, Predoctoral Fellow
- Alexander Sviridov, PhD, Guest Researcher
- Zachary Ulissi, Summer Student
- Laleh Najafizadeh, PhD, Postdoctoral Fellow
- Rizwan Jagani, Summer Student
- Atieh Bakhtiar, Summer Student
- Krishna Nagineni, Summer Student
- Michael Kellman, Summer Student
- Vipin Reddy, Summer Student
Our objectives are to devise quantitative biophotonics methodologies and associated instrumentations in order to study 1) biological phenomena at different length scales—from nanoscopy to microscopy—and 2) diffuse biophotonics. We take advantage of our expertise in stochastic modeling to study complex biological phenomena whose properties are characterized by elements of randomness in both time and space, such as light-tissue interactions. We explore various properties of light-matter interactions as sources of optical contrasts, such as polarization properties, endogenous or exogenous fluorescent labels, absorption (e.g., hemoglobin or chromophore concentration), and/or scattering. We have used these contrast mechanisms for tomographic and spectroscopic methods to develop benchtop instrumentation for preclinical and clinical uses. We are identifying physiological sites where optical techniques might be clinically practical and offer new diagnostic knowledge and/or less morbidity than existing diagnostic methods.
Quantitative characterization of tissue
The fibrous nature of many biological tissues, such as collagen, muscle, and teeth, imparts optically anisotropic properties to these tissues. Resulting changes in the polarization state of the backscattered light may provide additional opportunities for optical imaging, aimed at characterization and early diagnosis of diseases. Applying illumination to the tissue by a linearly polarized light and measuring intensity distributions of co- and cross-polarized components of backscattered photons (the latter coming from deeper tissue layers) allows one to analyze subsurface hidden structures that are not accessible for conventional visualization. However, to take full advantage of this approach to quantify structures' characteristic scales and directionality, sophisticated statistical methods of data processing must be applied.
We developed an algorithm, based on Pearson correlation analysis, that allows one, for example, to observe and quantify early stages of skin fibrosis. Taking into account that the uterine cervix is composed of muscle and collagen, we designed a novel polarization adapter for a conventional colposcope. The idea is to quantify structural changes, related to cervix ripening, from the cross-polarized images of the cervix. Phantom experiments have demonstrated the ability of the designed system to visualize the collagen network at the scale of 10 to 20 µ. One potential application of the methodology that we are now pursuing is for characterizing the state of the uterus to predict preterm birth. In collaboration with physicians, we designed a clinical protocol to collect and analyze the in vivo data, optimizing their potential diagnostic content.
Preclinical studies on fluorescence imaging of HER2-positive breast cancer
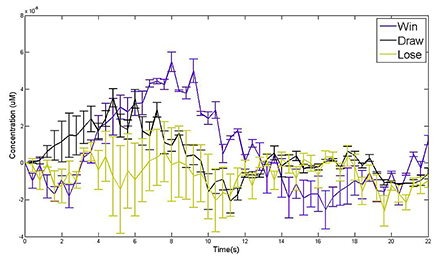
Figure 1
Typical mouse with tumor and intensity image after injecting Affibody-Alexa Fluor 750 to target HER2 receptors. (click image to enlarge)
Development of fluorescently labeled cell-surface markers has led to a revolution in specific molecular diagnosis of disease by histopathology and contributed to our understanding of the molecular origins of disease processes. Highly specific exogenous markers are now being designed that target given receptor sites in the body. Fluorophores conjugated to the engineered molecules preferentially concentrate at the sites of interest after injection (see for example, Figure 1, presenting our results on fluorescence imaging of a HER2-positive tumor in a mouse model; a bright spot in the image to the right corresponds to accumulation of the fluorophore in the tumor area). Such concentration parallels the delivery of some novel drugs, e.g., trastuzumab, to the diseased site and opens a new era of the "treat and image" paradigm.
HER2 overexpression in breast cancers is associated with poor prognosis and resistance to therapy. Current techniques for estimating this important characteristic use ex vivo assays that require tissue biopsies. We have suggested a novel noninvasive method to characterize HER2 expression in vivo with optical imaging and HER2-specific probes that could be used concomitantly with HER2-targeted therapy. In collaboration with Jacek Capala, we developed fluorescent markers, based on HER2-specific affibody molecules, for in vivo monitoring of the level of overexpression of HER2, and started pre-clinical studies in a mouse model. Analysis of the imaging parameters, estimated from the sequence of optical images, revealed a correlation between tumor cell amplification/overexpression of HER2 and these parameters. For example, experimental data for xenografts of BT474 indicated that the initial accumulation rate of the contrast agent in the tumor depends linearly on the HER2 expression, as measured ex vivo by an ELISA assay for the same tumor. Results obtained from tumors expressing various levels of HER2 substantiate a similar relationship between the initial slope and amplification/overexpression of HER2. Optical imaging, combined with mathematical modeling, might allow noninvasive monitoring of HER2 expression in vivo. As early reporters of downregulation of receptors in response to monoclonal antibodies, the developed probes show promise for in vivo monitoring for HER2-based chemotherapy.
Fluorescence lifetime imaging
Time-resolved fluorescence imaging allows us, in principle, to probe conditions in the vicinity of the fluorophore. This task requires specially designed fluorophores, sensitive to pH, temperature, etc. In collaboration with Samuel Achilefu and Gary Griffiths, we are working on the development of probes whose fluorescence lifetime is sensitive to pH; such probes can provide additional diagnostic information. Preliminary results indicate that one of the developed dyes possesses this property in the clinically important range of pH 5.5-7 (in normal tissue, pH is 7 to 7.5, but many solid tumors exhibit a pH value that can be as low as 5.6).
We are using our in-house small-animal imaging system to develop more accurate methods of 3-D localization of the fluorophores inside turbid media. Experiments with tissue-like phantoms substantiate the linear dependence of the position of the maximum (peak point) in time-of-flight intensity distribution of the emitted light on the fluorophore depth, a relationship that can be used in reconstruction algorithms. On the basis of a random walk model, we developed a general analytical model, describing time-resolved fluorescence from small fluorophores deeply embedded within tissue, and successfully applied it for reconstruction of intrinsic fluorescence lifetime.
Structural and functional brain imaging
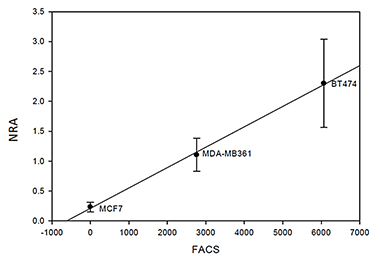
Figure 2
A) Portable fNIRS System. The system consists of a sensor pad and an acquisition box. FNIRS data are visualized and recorded on a laptop computer. B) fNIRS Pad. The sensor pad is composed of 4 light sources and 10 detectors with a 16-points configuration system. C) fMRI Results (Krueger F, et al., In: Banich, MT, ed. Cognitive Neuroscience and Neuropsychology 2009;1093). The complexity judgment task revealed activation in the frontopolar cortex. D) fNIRS Results. Sample data for one subject shows an increase of 10% in blood flow at the location identified by Krueger et al. during performance of the same task (values in color bar represent ratio of light intensity). (click image to enlarge)
Recent years have seen the arrival of near-infrared imaging as a potential tool in clinical assessment of hemodynamics in tissue. One key developing area for imaging is functional brain imaging with Diffuse Optical Tomography (DOT). Another area of interest has been the evolution of optical tools to detect functional anomalies in the human head; these include vascular problems in neonates and potential-stroke imaging in adults. In Figure 2, we illustrate a light field generated on an adult head model, meshed from an MRI scan. This field (from the source) is then combined with the adjoint field (from a detector) to give the Photon Measurement Density Function (PMDF), or sensitivity to change in optical properties, showing how light accesses information from the cortex. Many overlapping PMDF's can be used to reconstruct the volumetric optical properties, giving rise to the map of hemodynamic response.
We have been investigating how to move near-infrared functional imaging (NIRFI) into common use. It is limited by a variety of factors, but most by pre-existing techniques such as fMRI. In our work, we have identified two key patient groups—hemispherectomy patients and penetrating traumatic brain injury (TBI) patients—for whom fMRI cannot easily be used. This has created an opening for NIRFI use, but has required the development of new approaches. In both cases we must pay attention to the underlying changes in the photo physics away from the classical diffusion approximation. To handle these cases, we developed appropriate models and are working to improve quantitation. Further, we developed a novel, level sets–based algorithm that enhances both quantitation and localization in DOT.
Multispectral imaging
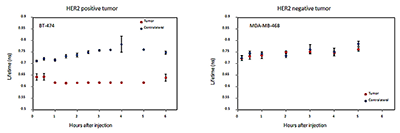
Figure 3
Multi-modality imaging of a healthy volunteer's arm, showing a reconstructed blood volume map combined with an OCT structural image. (click image to enlarge)
We continued the multispectral imaging project to monitor non-invasively the efficacy of treating Kaposi's sarcoma (K-S) lesions. The focus of this project was to fully quantify skin chromophore concentrations. In Figure 3, we present an example of a K-S image, as seen in the total blood volume map. To make our quantification accurate, we took into account the image distortions due to curved shape of imaged object (e.g., forearm); the curvature biases the acquired intensity distributions. To remove this artifact, we developed a curvature-correction algorithm, which removes any intensity variation due to shape and recovers the desired data signal over the entire image area. The algorithm is based on either averaging of rows and columns of the image to remove the data signal and extract the curvature or on fitting of a polynomial function for all rows and columns. We have analyzed the limitations of both methods. We performed numerical simulations, phantom experiments, and in vivo experiments and showed that real intensity values can be recovered within a 1.2% error and that a pixel-wise assessment of skin chromophores over the entire image area is possible. Without the curvature correction, the shape of the object led to intensity variations, which caused results of the reconstruction to be over- or underestimated (low vs. high angular dependence).
Publications
- Sviridov AP, Ulissi Z, Chernomordik V, Hassan M, Boccara AC, Gandjbakhche AH. Compact polarization camera with liquid-crystal retarder for patterning of biological textures. Biomedical Optics 2008;OSA Technical Digest, Paper BTuF48.
- Lee SB, Hassan M, Fisher R, Chertov O, Chernomordik V, Marek GK, Gandjbakhche A, Capala J. Affibody molecules for in vivo characterization of HER2-positive tumors by near-infrared imaging. Clin Cancer Res. 2008;14:3840-3849.
- Binzoni T, Vogel A, Gandjbakhche A, Marchesini R. Detection limits of multi-spectral optical imaging under the skin surface. Phys Med Biol. 2008;53:617-636.
- O'Mahony D, Gandjbakhche AH, Hassan M, Vogel A, Yarchoan R. Imaging techniques for Kaposi's sarcoma. J HIV Ther. 2008;13:65-71.
- Berezin MY, Guo K, Teng B, Edwards WB, Anderson CJ, Vasalatiy O, Gandjbakhche A, Griffiths GL, Achilefu S. Radioactivity-synchronized fluorescence enhancement using a radionuclide fluorescence-quenched dye. J Am Chem Soc. 2009;131:9198-9200.
Collaborators
- Samuel Achilefu, PhD, Washington University School of Medicine, St. Louis, MO
- Franck Amyot, PhD, Cognitive Neuroscience Section, NINDS, Bethesda, MD
- Robert Balaban, PhD, Laboratory of Cardiac Energetics, NHLBI, Bethesda, MD
- Tiziano Binzoni, PhD, Université de Genève, Geneva, Switzerland
- Claude Boccara, PhD, École Supérieure de Physique et de Chimie Industrielles, Paris, France
- Kevin Camphausen, PhD, Radiation Oncology Branch, NCI, Bethesda, MD
- Jacek Capala, PhD, Radiation Oncology Branch, NCI, Bethesda, MD
- Christian Combs, PhD, Light Microscopy Facility, NHLBI, Bethesda, MD
- Stavros Demos, PhD, Lawrence Livermore National Laboratory, Livermore, CA
- Israel Gannot, PhD, Tel Aviv University, Tel Aviv, Israel, and The George Washington University, Washington, DC
- Jordan Grafman, PhD, Cognitive Neuroscience Section, NINDS, Bethesda, MD
- Gary Griffiths, PhD, Imaging Probe Development Center, NIH, Rockville, MD
- Jeremy Hebden, PhD, University College London, London, UK
- Ilko Ilev, PhD, Office of Science and Engineering Laboratories, FDA, Bethesda, MD
- Jay Knutson, PhD, Laboratory of Molecular Biophysics, NHLBI, Bethesda, MD
- Sang Bong Lee, PhD, Radiation Oncology Branch, NCI, Bethesda, MD
- Ralph Nossal, PhD, Program on Physical Biology, NICHD, Bethesda, MD
- Anne Plant, PhD, NIST, Gaithersburg, MD
- Dan Sackett, PhD, Program on Physical Biology, NICHD, Bethesda, MD
- Alexsandr Smirnov, PhD, Light Microscopy Facility, NHLBI, Bethesda, MD
- Paul Smith, PhD, Division of Bioengineering and Physical Science, ORS, Bethesda, MD
- Eric Wassermann, MD, Cognitive Neuroscience Section, NINDS, Bethesda, MD
- Ronald Waynant, PhD, Office of Science and Engineering Laboratories, FDA, Bethesda, MD
- George Weiss, PhD, Division of Computational Bioscience, CIT, NIH, Bethesda, MD
- Robert Yarchoan, MD, HIV and AIDS Malignancy Branch, NCI, Bethesda, MD
- Rafal Zielinski, PhD, Radiation Oncology Branch, NCI, Bethesda, MD
Contact
For more information, email amir@helix.nih.gov.