You are here: Home > Section on Analytical and Functional Biophotonics
Quantitative Biophotonics for Tissue Characterization and Function
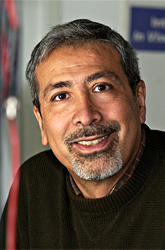
- Amir H. Gandjbakhche, PhD, Head, Section on Analytical and Functional Biophotonics
- Victor Chernomordik, PhD, Staff Scientist
- Jason Riley, PhD, Research Fellow
- Yasaman Ardeshirpour, PhD, Postdoctoral Fellow
- Jana Kainerstorfer, PhD, Postdoctoral Fellow
- Laleh Najafizadeh, PhD, Postdoctoral Fellow
- Franck Amyot, PhD, Guest Researcher
- Alexander Sviridov, PhD, Guest Researcher
- Afrooz Azari, MS, Predoctoral Student
- Nader Shahni Karamzadeh, Volunteer
- Fatima A. Chowdhry, MD, Volunteer
- Atieh Bakhtiar, Volunteer
Our objectives are to devise quantitative biophotonics methodologies and associated instrumentations in order to study biological phenomena at different length scales—from nanoscopy to microscopy—and diffuse biophotonics. We take advantage of our expertise in stochastic modeling to study complex biological phenomena whose properties are characterized by elements of randomness in both time and space, such as light-tissue interactions. We explore various properties of light-matter interactions as sources of optical contrasts, such as polarization properties, endogenous or exogenous fluorescent labels, absorption (e.g., hemoglobin or chromophore concentration), and/or scattering. We used these contrast mechanisms for tomographic and spectroscopic methods to develop benchtop instrumentation for preclinical and clinical uses. We are identifying physiological sites where optical techniques might be clinically practical. Such techniques may offer new diagnostic knowledge and/or less morbidity than existing diagnostic methods.
Structural and functional brain imaging
Functional near-infrared spectroscopy (fNIRS) is a useful tool for neuroimaging studies. In the case of traumatic brain injuries (TBI), lack of sensitive bedside tests for executive function can make it difficult to asses frontal lobe involvement, which may not even be suspected in survivors who are otherwise neurologically intact. Thus, casualties with occult frontal impairment and deficits in judgment may be discharged inappropriately. There is, therefore, a need for reliable tests of frontal lobe function that can be administered quickly by individuals without neuropsychological training. In this project, we are using two parametric paradigms (the n-back working memory task and the judgment of complexity) to measure the relationship between the loads or difficulties of the task and the percentage blood flow change. Our hypothesis is this relationship differs between a TBI population and a healthy group. We also looked for the association between stimulus complexity and the magnitude of blood-flow increase in the frontopolar cortex. For each experiment, we co-registered all channels with the MRI anatomical patient image using a stereotactic camera. For the group study, we found activation at the coordinates x = −7, y = 85, z = 13 in the MNI (Montreal Neurological Insitute) atlas, which is consistent with results from functional magnetic resonance imaging (fMRI) studies. We also looked for the association between stimulus complexity and the magnitude of blood-flow increase in the frontopolar cortex. The second paradigm is the working memory test (the n-back task). We showed that the load of the working memory task is directly related with the intensity of the activation. From the subject measurements, we constructed a normative database for simple cognitive tests that can be useful in evaluating cognitive disability such as mild TBI. The second step is to test our hypothesis in a TBI population. A collaborative protocol with Ramon Diaz-Arrastia has been accepted, and we expect to recruit TBI patients at the end of 2012.
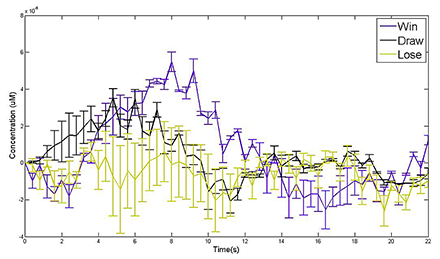
Click image to enlarge.
Figure 1. Changes of blood oxygenation in motor cortex during "Rock-Paper-Scissor" competitive game
The subject is seated in front of a monitor displaying a video of one of the nine possible solutions of the competitive game. Each solution is displayed three times. We measure the change in microM of oxyhemoglobin concentration in the motor cortex for three outcomes (Win, Draw or loose).
A separate part of the fNIRS project is related to our bench-to-beside award—"fNRIS imaging for Identification of Neural Markers for Autistic Spectrum Disorder (ASD)." In this project, we identified two paradigms that can give different outcomes depending of the population (healthy vs. ASD). The first paradigm is a well establish fMRI paradigm—the "go and no go task." Preliminary results show activation in the dorsolateral prefrontal cortex (DLPFC) in healthy population, as expected. The other original paradigm is a competitive game more commonly known as "rock-paper-scissor." To watch a competitive game we rely on our ability to empathize and relate to the internal motor and sensory states of others. The system that allows us to do this is a network called the mirror neuron system (MNS). The network supports understanding actions of others by generating resonance behavior–neural activity during actions as well as while observing the actions of others. Earlier studies concluded that the MNS runs through several areas of the brain, including the motor cortex. The motor cortex is important for both planning and carrying out actions. We examined the response of the MNS in the motor cortex while observing a two-player competitive game. We found that (Figure 1) the responses depend on the outcome of the game (WIN, LOSE, DRAW) and the spatial relationship between the subject and the presented model.
Children with ASDs often display deficits in their ability to empathize with others and relate to the actions of others. Evidence suggests that this may be the result of a dysfunctional MNS. We posit that data produced from studies on 'healthy subjects' will differ from data collected in subjects with ASDs.
Another brain imaging project also uses a near infrared spectroscopy device but looks at changes in space rather than time. The aim is to use the instrumental motion as a signal. As a practical application to demonstrate this approach, we designed a novel NIR hematoma detection device. The device is based on a simplified single-source configuration with a dual separation detector array and uses motion as a signal for detecting changes in blood volume in the dural regions of the head.
The rapid triage of hematomas required in an emergency room will necessitate use of more sophisticated/expensive imaging facilities such as CT/MRI units. We performed experiments on a phantom for simulation purposes. Our phantom is a uniform resin cylinder containing three small inclusions of different optical properties (scatter and absorption). The results demonstrate excellent localization of inclusions as well as good quantitative comparisons. Future work will involve building a statistical model of hematomas based on existing CT data. This approach will allow us to optimize the design of a device for the detection of both unilateral and bilateral hematomas.
Preclinical studies on fluorescence imaging of HER2–positive breast cancer
Fluorescently labeled cell-surface markers contributed to a rapid advancement in specific molecular diagnosis of diseases. The engineered molecules can target cell receptors, important for diagnostics and choice of therapy. Fluorescence imaging with a receptor-specific probe permits mapping the tissue abnormality and characterizing the disease. One important example of a cell receptor that plays an important role in aggressive tumor behavior is a well known biomarker, human epidermal growth factor receptor type 2 (HER2). Its overexpression is associated with poor clinical outcome. Current techniques for estimating HER2 receptor expression use ex vivo assays that require tissue biopsies, which are not compatible with continuous monitoring of the response of the tumor receptors to therapy. Thus, non-invasive in vivo methods would be preferable, especially at early stages of disease. We developed a novel noninvasive method to characterize HER2 expression in vivo, using optical imaging and involving HER2–specific probes that could be administered simultaneously with HER2–targeted therapies.
Our approach is based on application of small HER2–specific Affibody molecules, tagged with a fluorescent dye. We used an in-house time-resolved near-infrared fluorescence imaging system in combination with a novel method to analyze the data to characterize in vivo tumors with varying levels of HER2 overexpression in the cancer cells. Initial tests of HER2–specific Affibody, labeled by the fluorophore DyLight 750, in a mouse model of breast carcinoma showed that HER2 overexpression can be estimated from an analysis of a time series of tumor fluorescence images, if the probe pharmacokinetics is taken into account by a ligand-receptor kinetic model.
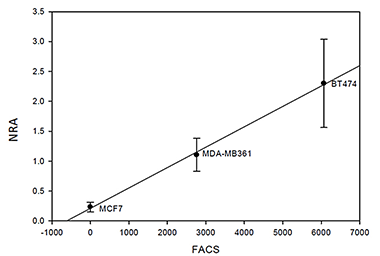
Click image to enlarge.
Figure 2. Non-invasive quantification of HER2 receptors in different cancer cell lines is highly correlated with FACS measurements.
Relationship between normalized rates of accumulation (NRA) of the HER2–specific Affibody probe and HER2 expression in three different tumors.
We applied the ligand-receptor kinetic model to characterize HER2 expression in three breast cancer xenograft models, expressing different levels of HER2. HER2–specific Affibody conjugated to DyLight750 was injected intravenously. Based on pharmacokinetic studies, we calculated average values of Normalized Rate of Accumulation (NRA) for each tumor type and compared them with the Affibody-DyLight-488 retention measured by flow cytometry (FACS). The results indicate a good linear correlation between both parameters over the broad range of HER2 overexpression in cancer cells, substantiating our idea that in vivo fluorescence imaging can provide quantitative information on the HER2 overexpression (Figure 2). In the same set of experiments, we investigated the lifetime of fluorescent probes as a marker of tumor environment. As a first step, we demonstrated in live animals that the fluorescence lifetime can be used to detect the binding of targeted optical probes to extracellular receptors on cancer cells in vivo. For this study, the HER2–specific Affibody probe conjugated to Dylight 750 was injected intravenously in mice bearing HER2–positive and HER2–negative tumors, which were then imaged with the time-resolved imaging system. Our results show that binding of the specific probe to HER2 receptors in our model system results in a noticeable reduction in fluorescence lifetimes (Figure 3). Thus, lifetime measurements can be used as a specific in vivo indicator of the receptor binding process and, correspondingly, HER2 expression levels, especially for early evaluation of the efficacy of therapy.
Figure 3. Lifetime of imaging probe can be used to quantify probe-receptor binding.
Fluorescence lifetime at the tumor and the contralateral site of breast carcinoma xenografts with (left) high level of HER2 expression (right) no-HER2 expression
Multispectral imaging
The oncology community is testing several novel targeted approaches for the treatment of a variety of cancers. With regard to monitoring vasculature, it is desirable to develop and assess noninvasive and quantitative techniques that can not only monitor structural changes but can also assess the functional characteristics or the metabolic status of the tumor. We are investigating three potential noninvasive imaging techniques to monitor patients undergoing experimental therapy: infrared thermal imaging (thermography), laser Doppler imaging (LDI), and multi-spectral imaging. We are testing the imaging techniques on subjects with Kaposi's sarcoma (KS), a highly vascular tumor that occurs frequently in patients infected with HIV. Cutaneous KS lesions are easily accessible for noninvasive techniques to image tumor vasculature and thus represent a tumor model in which to assess parameters of angiogenesis. We are pursuing the KS studies in ongoing clinical trials under four different NCI protocols. We showed that our multi-modality techniques can non-invasively monitor the functional properties of the tumor and surrounding tissues and have the potential to predict treatment outcomes. We recently obtained angiogenic markers (blood volume and oxygenation) in real time. Using novel data analysis tools based on Principal Component Analysis, we showed that a KS lesion can be imaged and the functional state of the tumor assessed in real time. In combination with optical coherence tomography (OCT), we recently obtained quantitative measures of blood volume and oxygenation in real time. We also found that quantitative results can be obtained if the underlying skin structures are taken into account. In order to obtain those structures, we developed a spectral domain OCT system that provides 3D images of the skin.
Polarization imaging
Polarization imaging is a promising tool to visualize structures hidden below the tissue surface. Analysis of structures such as the collagen network can be used to assess the possible transition from normal tissue function to diseased tissue. We developed sophisticated techniques of data analysis, based on the Pearson correlation procedure, that permit enhancement of image quality and that reveal regions of high statistical similarities within the noisy images, enabling characterization of subsurface structural features of biological tissues. To realize the potential of the method, we designed a user-friendly polarization imaging system that simultaneously images cross- and co-polarized light. Preliminary experiments demonstrated that the designed polarization adapter in combination with data analysis algorithms can provide quantitative information on collagen structure. We incorporated this adapter into a conventional colposcope and started a clinical protocol to study tissue structures in the cervix. We collected data on 10 healthy volunteers at luteal and follicular phases of the cycle and are analyzing them to optimize the system and update the clinical protocol.
Additional Funding
- Bench to Bedside Award
- Center for Neuroscience and Regenerative Medicine
- NIBIB
Publications
- Ardeshirpour Y, Chernomordik V, Capala J, Hassan M, Zielinsky R, Griffiths G, Achilefu S, Smith P, Gandjbakhche A. Using in-vivo fluorescence imaging in personalized cancer diagnostics and therapy, an Image and Treat Paradigm. TCRT 2011;10(6):549-560.
- Zielinski R, Lyakhov I, Hassan M, Kuban M, Shafer-Weaver K, Gandjbakhche AH, Capala J. HER2-Affitoxin: a potent therapeutic agent for the treatment of HER2-overexpressing tumors. Clin Cancer Res 2011;17(15):5071-5081.
- Ardeshirpour Y, Zielinski R, Chernomordik V, Capala J, Griffiths G, Vasalatiy O, Achilefu S, Gandjbakhche A, Hassan M. In vivo fluorescence lifetime imaging monitors binding of specific probes to cancer biomarkers. PLoS ONE 2012;7(2):e31881.
- Riley JD, Amyot F, Pohida T, Pursley R, Ardeshipour Y, Kainerstorfer JM, Najafizadeh L, Chernomordik V, Smith P, Smirniotopoulos J, Wassermann EM, Gandjbakhche AH. A hematoma detector—a practical application of instrumental motion as signal in near infra-red imaging. Biomed Optics Express 2012;3(1):192-205.
- Amyot F, Zimmermann T, Riley J, Kainerstorfer JM, Chernomordik V, Mooshagian E, Najafizadeh L, Krueger F, Gandjbakhche AH, Wassermann EM. Normative database of judgment of complexity task with functional near infrared spectroscopy--application for TBI. Neuroimage 2012;60(2):879-883.
Collaborators
- Samuel Achilefu, PhD, Washington University School of Medicine, St. Louis, MO
- Robert Balaban, PhD, Laboratory of Cardiac Energetics, NHLBI, Bethesda, MD
- Claude Boccara, PhD, École Supérieure de Physique et de Chimie Industrielles, Paris, France
- Kevin Camphausen, PhD, Radiation Oncology Branch, NCI, Bethesda, MD
- Jacek Capala, PhD, Radiation Oncology Branch, NCI, Bethesda, MD
- Christian Combs, PhD, Light Microscopy Facility, NHLBI, Bethesda, MD
- Stavros Demos, PhD, Lawrence Livermore National Laboratory, Livermore, CA
- Ramon Diaz-Arrastia, MD, PhD, Uniformed Services University of the Health Sciences, Bethesda, MD
- Israel Gannot, PhD, Tel Aviv University, Tel Aviv, Israel, and The George Washington University, Washington, DC
- Gary Griffiths, PhD, Imaging Probe Development Center, NIH, Rockville, MD
- Alan Halpern, MD, Memorial Sloan-Kettering Cancer Center, New York, NY
- Ilko Ilev, PhD, Office of Science and Engineering Laboratories, FDA, Bethesda, MD
- Jay Knutson, PhD, Laboratory of Molecular Biophysics, NHLBI, Bethesda, MD
- Ralph Nossal, PhD, Program in Physical Biology, NICHD, Bethesda, MD
- Sinisa Pajevic, PhD, Division of Computational Bioscience, CIT, NIH, Bethesda, MD
- Tom Pohida, MS, Division of Computational Bioscience, CIT, NIH, Bethesda, MD
- Dan Sackett, PhD, Program in Physical Biology, NICHD, Bethesda, MD
- Alexsandr Smirnov, PhD, Light Microscopy Facility, NHLBI, Bethesda, MD
- Paul Smith, PhD, Division of Bioengineering and Physical Science, Office of Research Services, NIH, Bethesda, MD
- Eric Wassermann, MD, Cognitive Neuroscience Section, NINDS, Bethesda, MD
- Robert Yarchoan, MD, HIV and AIDS Malignancy Branch, NCI, Bethesda, MD
- Rafal Zielinski, PhD, Radiation Oncology Branch, NCI, Bethesda, MD
Contact
For more information, email amir@helix.nih.gov.