You are here: Home > Section on Cellular and Synaptic Physiology
Hippocampal Interneurons and Their Role in the Control of Network Excitability
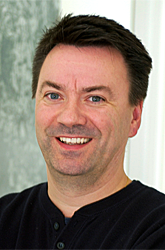
- Chris J. McBain, PhD, Head, Section on Cellular and Synaptic Physiology
- Christian Cea del Rio, BS, Graduate Student
- Michael Daw, PhD, Visiting Fellow
- Brian Erkkila, PhD, Visiting Fellow
- Tsz-wan Michelle Ho, BS, Graduate Student
- Brian Jefferies, BS, Biologist
- Elizabeth Mayne, BSc, Graduate Student
- Kenneth Pelkey, PhD, Staff Scientist
- Madhav Sukumaran, BSc, Graduate Student
- Christine Torborg, PhD, Visiting Fellow
- Ludovic Tricoire, PhD, Visiting Fellow
- Xiaoqing Yuan, MSc, Biologist
- Sean Barron, PhD, Postdoctoral Fellow
- Jose Matta, Visiting Fellow
GABAergic inhibitory interneurons constitute a population of hippocampal neurons whose high degree of anatomical and functional divergence make them suitable candidates for controlling the activity of large populations of principal neurons. Interneurons (INT) receive strong excitatory glutamatergic innervation via numerous anatomically distinct afferent projections; recent evidence has revealed that the molecular composition of the AMPA-preferring class of glutamate receptors expressed at INT synapses is often distinct from that of receptors found at principal cell synapses. Our goal is to identify the types of ligand-gated ion- and voltage-gated ion channels and the roles they play in controlling the cortical network. Specifically, we strive to understand the functions of these receptors in regulating synaptic and neuronal excitability, short- and long-term plasticity, and the development and pathophysiology of the mammalian cortical structure. Through this research, we envision a better understanding of the specific mechanisms regulating excitability in precise cohorts of central nervous system cells and thus more targeted and valid methods of treatment of a wide array of central nervous system disease states.
Narp regulates homeostatic scaling of excitatory synapses on parvalbumin interneurons.
Homeostatic synaptic scaling alters the strength of synapses to compensate for prolonged changes in network activity and involves both excitatory and inhibitory neurons. The immediate-early gene Narp (neuronal activity–regulated pentraxin) encodes a secreted synaptic protein that can bind to and induce clustering of AMPA receptors (AMPARs). We found that Narp prominently accumulated at excitatory synapses on parvalbumin-expressing interneurons (PV-INs). Increasing network activity resulted in a homeostatic increase of excitatory synaptic strength onto PV-INs that enhanced inhibitory drive—a response that was absent from neurons cultured from Narp−/− mice. Activity-dependent changes in the strength of excitatory inputs on PV-INs in acute hippocampal slices were also dependent on Narp, and Narp−/− mice had elevated sensitivity to kindling-induced seizures. We propose that Narp recruits AMPARs at excitatory synapses onto PV-INs to rebalance network excitation/inhibition dynamics following episodes of increased circuit activity.
Control of CA3 output by feedforward inhibition despite developmental changes in the excitation-inhibition balance
In the somatosensory cortex, the relative balance of excitation and inhibition determines how effectively feedforward inhibition enforces the temporal fidelity of action potentials (APs). Within the CA3 region of the hippocampus, glutamatergic mossy fiber (MF) synapses onto CA3 pyramidal cells (PCs) provide strong monosynaptic excitation that exhibits prominent facilitation during repetitive activity. We demonstrated in the juvenile CA3 that MF-driven polysynaptic IPSCs facilitate to maintain a fixed EPSC-IPSC ratio during short-term plasticity. In contrast, in young adult mice, this MF-driven polysynaptic inhibitory input can facilitate or depress in response to short trains of activity. Transgenic mice lacking the feedback inhibitory loop continue to exhibit both facilitating and depressing polysynaptic IPSCs, indicating that this robust inhibition is cannot be attributed to the secondary engagement of feedback inhibition. Surprisingly, eliminating MF-driven inhibition onto CA3 pyramidal cells by blockade of GABAA receptors did not lead to a loss of temporal precision of the first AP observed after a stimulus but, in many cases, triggered a long, excitatory plateau potential capable of triggering repetitive AP firing. The observations indicate that, unlike in other regions of the brain, the temporal precision of single MF-driven APs is dictated primarily by the kinetics of MF EPSPs, not feedforward inhibition. Instead, feedforward inhibition provides robust regulation of CA3 PC excitability across development to prevent excessive depolarization by the monosynaptic EPSP and multiple AP firings.
Presynaptic kainate receptor activation preserves asynchronous GABA release despite the reduction in synchronous release from hippocampal CCK interneurons.
Chemical synaptic transmission classically occurs when neurotransmitter is released from a presynaptic terminal in response to an AP in a tightly time-locked fashion. Neurotransmitter release at most central synapses is synchronized to the timing of presynaptic APs. However, it has been shown at several central synapses that, in addition to synchronous release, some transmitter may be released in a longer and less precise time window such that release is asynchronous and not locked to individual presynaptic triggers. We showed that three classes of DSI (depolarization-induced suppression of inhibition) –expressing, presumed cholecystokinin (CCK) -containing, hippocampal interneurons show highly asynchronous release in response to trains of APs. This asynchrony is correlated with the class of presynaptic interneuron but is unrelated to their postsynaptic cell target. Asynchronous (from CCK-containing interneurons) release and synchronous (from parvalbumin-containing interneurons) release show a near identical calcium dependence, suggesting a divergence of release mechanisms downstream of the calcium sensor. Asynchronous IPSCs include very large (up to 500 pA/7nS) amplitude events, which persist in low extracellular calcium and strontium, showing that the events result from quantal transmitter release at single release sites. The fact that asynchronous release from CCK-containing interneurons is a widespread phenomenon indicates a fundamental role for these cells within the hippocampal network that is distinct from the phasic inhibition provided by PV-containing interneurons.

Figure 1. Interneurons in the GAD-65 GFP mouse
Numerous subpopulations are present within a hippocampal section derived from a GAD-65 GFP transgenic mouse.
In a second followup study, we demonstrated that activation of presynaptic kainate receptors (KARs) reduces inhibitory transmission. Using paired recordings, we show that KAR activation causes a reduction in presynaptic release from CCK- but not PV-containing interneurons and that this reduction is observed when pyramidal cells, but not interneurons, are the postsynaptic target. We also show that, although the synchronous release component is reduced, the barrage of asynchronous GABA release from CCK interneurons during sustained firing is unaffected by KAR activation. This indicates that presynaptic KARs preserve and act in concert with asynchronous release to switch CCK interneurons from a phasic inhibition mode to produce prolonged inhibition during periods of intense activity.
M3 muscarinic acetylcholine receptor expression confers differential cholinergic modulation to neurochemically distinct hippocampal basket cell subtypes.
Cholinergic neuromodulation of hippocampal circuitry promotes network oscillations and facilitates learning and memory through cellular actions on both excitatory and inhibitory circuits. Despite widespread recognition that neurochemical content discriminates between functionally distinct interneuron populations, there has been no systematic examination of whether neurochemically distinct interneuron classes undergo differential cholinergic neuromodulation in the hippocampus. Using GFP transgenic mice that permit the visualization of perisomatically targeting PV-positive or CCK-positive basket cells (BCs), we tested the hypothesis that neurochemically distinct interneuron populations are differentially engaged by muscarinic acetylcholine receptor (mAChR) activation. Cholinergic fiber activation revealed that CCK-BCs were more sensitive to synaptic release of ACh than PV-BCs. In response to depolarizing current steps, mAChR activation of PV-BCs and CCK-BCs also elicited distinct cholinergic response profiles, differing in mAChR-induced changes in AP waveform, firing frequency, and intrinsic excitability. In contrast to PV-BCs, CCK-BCs exhibited a mAChR-induced afterdepolarization (mADP) that was frequency- and activity-dependent. Pharmacological, molecular, and loss-of-function data converged on the presence of M3 mAChRs in distinguishing CCK-BCs from PV-BCs. Firing frequency of CCK-BCs was controlled through M3 mAChRs but PV-BC excitability was altered solely through M1 mAChRs. Lastly, upon mAChR activation, glutamatergic transmission enhanced cellular excitability preferentially in CCK-BCs but not in PV-BCs. Our findings demonstrate that cell type–specific cholinergic specializations are present on neurochemically distinct interneuron subtypes in the hippocampus, revealing an organizing principle such that cholinergic neuromodulation depends critically on neurochemical identity.
Cholinergic modulation amplifies the intrinsic oscillatory properties of CA1 hippocampal cholecystokinin-positive interneurons.
CCK-containing interneuron subpopulations provide GABAergic inhibition to multiple surface domains of CA1 hippocampal pyramidal cells. CCK-BCs control AP initiation through perisomatic inhibition, whereas dendritically projecting Schaffer collateral–associated (SCAs) cells likely shape dendritic excitability and synaptic integration. We showed previously that mAChR activation regulates CCK-BCs firing properties and excitability; however, little is known about mAChR modulation of the related but anatomically distinct CCK-SCA population. Using whole-cell recordings and single-cell RT-PCR, we showed that muscarine elicited a biphasic hyperpolarizing-depolarizing voltage response in CCK-SCAs, which was mediated by opposing actions of M1 and M3 mAChRs respectively. In addition, like CCK-BCs, CCK-SCAs exhibited an M3-mediated increase in AP firing frequency and an after-depolarization mediated synergistically by both M1 and M3 mAChRs. Spontaneous M3-mediated membrane potential oscillations (1-2Hz) were observed in both CCK-SCAs and CCK-BCs. Using sinusoidal current injection, we examined how mAChR activation of CCK interneurons alters intrinsic oscillatory properties and AP frequency preference. In CCK-BCs and CCK-SCAs, APs occurred preferentially at delta/theta frequencies (1-7Hz) under control conditions. mAChR activation extended the AP phase-locking bandwidth into the theta and beta frequency range for CCK-SCAs and CCK-BCs, respectively; this was accompanied by changes in both AP phase and precision. In conclusion, anatomically distinct CCK+ cells show similar changes in excitability, firing pattern activity, and oscillatory preferences during cholinergic modulation suggesting that CCK+ interneurons likely act cooperatively to collectively synchronize the hippocampal network along the somatodendritic axis of the CA1 pyramidal cells during muscarinic receptor activation.
Common origins of nitric oxide synthase–expressing hippocampal Ivy and neurogliaform cells
GABAergic interneurons critically regulate cortical computation through exquisite spatiotemporal control over excitatory networks. Precision of this inhibitory control requires a remarkable diversity within interneuron populations that is largely specified during embryogenesis. Although interneurons expressing the neuronal isoform of nitric oxide synthase (nNOS) constitute the largest hippocampal interneuron cohort, their origin and specification remain unknown. Thus, as neurogliaform cells (NGC) and Ivy cells (IvC) represent the main nNOS interneurons, we investigated their developmental origins. Although considered distinct interneuron subtypes, NGCs and IvCs exhibited similar neurochemical and electrophysiological signatures, including NPY expression and late spiking. Moreover, lineage analyses, including loss-of-function experiments and inducible fate-mapping, indicated that nNOS IvCs and NGCs are both derived from medial ganglionic eminence (MGE) progenitors under the control of the transcription factor Nkx2-1. Surprisingly, a subset of NGCs lacking nNOS arises from caudal ganglionic eminence (CGE) progenitors. Thus, while nNOS NGCs and IvCs arise from MGE progenitors, a CGE origin distinguishes a discrete population of nNOS NGCs.
Additional Funding
- Dr. Torborg was the recipient of a PRAT Fellowship.
- Dr. Matta was the recipient of a PRAT Fellowship.
Publications
- Daw MI, Tricoire L, Erdelyi F, Szabo G, McBain CJ. Asynchronous transmitter release from CCK-containing inhibitory interneurons is widespread and target-cell independent. J Neurosci. 2009;29:11112-111122.
- Ho TW, Pelkey KA, Pelletier JG, Huganir RL, Lacaille J-C, McBain CJ. Burst firing induces postsynaptic LTD at developing mossy fiber-CA3 pyramid synapses. J Physiol. 2009;587:4441-4454.
- Chang MC, Park JM, Pelkey KA, Grabenstatter H, Xu D, Linden DJ, Sutula, TP, McBain CJ, Worley PF. Narp regulates homeostatic scaling of excitatory synapses on parvalbumin interneurons. Nat Neurosci. 2010;13:1090-1097.
- Tricoire L, Pelkey KA, Daw MI, Sousa VH, Miyoshi G, Jeffries B, Cauli B, Fishell G, McBain CJ. Common origins of hippocampal Ivy and nitric oxide synthase expressing neurogliaform cells. J Neurosci. 2010;30:2165-2176.
- Daw MI, Pelkey KA, Chittajallu R, McBain CJ. Presynaptic kainate receptor activation preserves asynchronous GABA release despite the reduction in synchronous release from hippocampal CCK interneurons. J Neurosci. 2010;30:11202-11209. Cea-del Rio CA, Lawrence JJ, Tricoire L, Erdelyi F, Szabo G, McBain CJ. M3 muscarinic acetylcholine receptor expression confers differential cholinergic modulation to neurochemically distinct hippocampal basket cell subtypes. J Neurosci. 2010;30:6011-6024.
Collaborators
- Bruno Cauli, PhD, Université Pierre et Marie Curie, Paris, France
- Gordon Fishell, PhD, New York University, New York, NY
- Gabor Szabo, PhD, Institute of Experimental Medicine, Budapest, Hungary
- Paul Worley, PhD, The Johns Hopkins University, Baltimore, MD
Contact
For more information, email mcbainc@mail.nih.gov or visit neuroscience.nih.gov/Faculty/Profile/chris-mcbain.aspx.