You are here: Home > Section on Nutrient Control of Gene Expression
Transcriptional and Translational Regulatory Mechanisms in Nutrient Control of Gene Expression
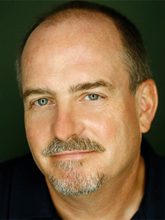
- Alan G. Hinnebusch, PhD, Head, Section on Nutrient Control of Gene Expression
- Nazanin Ashourian, BS, Graduate Student
- Wen-Ling Chiu, PhD, Postdoctoral Fellow
- Jinsheng Dong, PhD, Senior Research Assistant
- Naseem Gaur, PhD, Postdoctoral Fellow
- Cuihua Hu, BA, Special Volunteer
- Sebastien Lagiex, PhD, Postdoctoral Fellow
- Pilar Martin-Marcos, PhD, Postdoctoral Fellow
- Eun-Hee Park, PhD, Postdoctoral Fellow
- Hongfang Qiu, PhD, Staff Scientist
- Attiq Rehman, PhD, Postdoctoral Fellow
- Adesh Saini, PhD, Postdoctoral Fellow
- Fan Zhang, MS, Senior Research Assistant
- Fujun Zhou, PhD, Postdoctoral Fellow
- David Young, PhD, Postdoctoral Fellow
We study the regulation of amino acid biosynthetic genes in budding yeast as a means of dissecting molecular mechanisms of gene regulation at the translational and transcriptional levels. Transcription of these and many other genes is coordinately induced by the transcriptional activator Gcn4 in response to starvation for any amino acid. Expression of GCN4 is coupled to amino acid levels by a conserved translational control mechanism involving upstream open reading frames (uORFs) in GCN4 mRNA. Ribosomes translate the 5′-most uORF (uORF1), and, under non-starvation conditions, re-initiate translation at uORFs 2, 3, or 4 and then dissociate from the mRNA, keeping GCN4 translation repressed. In starvation conditions, the re-initiating ribosomes instead bypass uORFs 2–4 and re-initiate at GCN4, owing to decreased availability of the ternary complex (TC)—comprised of initiation factor 2 (eIF2), GTP, and initiator Met-tRNAi—which binds to the small (40S) ribosomal subunit to assemble a 43S pre-initiation complex (PIC). TC abundance is reduced in starved cells by phosphorylation of the alpha subunit of eIF2 (eIF2a) by Gcn2, a protein kinase conserved in all eukaryotes, converting eIF2 from substrate to inhibitor of its guanine nucleotide exchange factor (GEF) eIF2B. Hence, GCN4 translation is an in vivo indicator of impaired TC loading on 40S subunits. We previously exploited this fact to isolate mutations in subunits of eIF2B that constitutively de-repress GCN4 (Gcd− phenotype) by lowering TC assembly in the absence of eIF2 phosphorylation. More recently, we used the Gcd− selection to identify domains/residues in eIF1, eIF1A, and eIF3, as well as residues of 18S rRNA located near the "P" decoding site of the 40S subunit, all of which participate in rapid TC recruitment in vivo. In collaboration with Jon Lorsch's group, we demonstrated that segments/residues in eIF1, eIF1A, and 18S rRNA, which are implicated genetically in TC recruitment, also stimulate the same reaction in a fully reconstituted in vitro system.
We also investigate the roles of various eIFs and the 40S subunit in scanning the mRNA 5′ untranslated region and accurately identifying the AUG initiation codon. Our studies exploit a genetic selection for mutations that either elevate initiation at near-cognate UUG start codons (Sui− phenotype) or suppress this aberrant initiation event (Ssu− phenotype). In this way, we provided the first evidence that eIF1A and the c-subunit of eIF3 make critical contributions to accurate AUG recognition, and we localized these functions to the unstructured N- and C-tails of eIF1A and the N-terminal domain of eIF3c. In collaboration with Lorsch's group, we also provided strong evidence that dissociation of eIF1 from the PIC is a critical step in AUG recognition, indicating that this factor functions as a "gate-keeper" needed to suppress initiation at non–AUG triplets. Biochemical analysis revealed that eIF1 stabilizes an "open" conformation of the 40S subunit that is conducive to scanning and loading of the TC in a metastable state permitting inspection of successive triplets as they enter the P site for complementarity with the anticodon of Met-tRNAi. However, eIF1 must dissociate from the PIC for AUG selection, as the absence of eIF1 favors the closed, scanning-arrested conformation of the 40S subunit to which TC is more tightly bound. Our recent analysis of eIF1A provided strong evidence that 10 amino acid repeats in the C-terminal tail (dubbed "scanning enhancers", or SE elements) function together with eIF1 to stabilize the open complex and promote the first step of TC loading, but must then be ejected from the P site to achieve re-arrangement to the closed complex, with the TC more stably bound and Met-tRNAi base-paired with AUG. Furthermore, we identified scanning-inhibitory (SI) elements in the N-terminal tail and helical domain of eIF1A that oppose the SE elements and promote re-arrangement to the closed complex needed for AUG recognition.
In the arena of transcriptional control, we previously identified several co-activators required for gene activation by Gcn4 and defined the molecular program for recruitment of nucleosome-remodeling enzymes and adaptor proteins—proteins that remove repressive chromatin structure and recruit TATA-binding protein, other general transcription factors, and RNA polymerase II (Pol II) to promoters. We also demonstrated the co-transcriptional recruitment of the co-activator SAGA, containing histone acetyltransferase (HAT) subunit Gcn5, to transcribed coding sequences and implicated Spt-Ada-Gcn5 acetyltransferase (SAGA)/Gcn5 in transcription elongation. More recently, we showed that the histone H4 HAT complex NuA4 is also recruited co-transcriptionally to coding regions, in a manner stimulated by phosphorylation of Ser5 (Ser5P) in the heptad repeats of the Pol II C′-terminal domain (CTD) by the cyclin-dependent kinase Cdk7/Kin28, and we found that NuA4 association with nucleosomes further depends on H3 methylation, most likely attributable to chromodomains and a plant homeodomain (PHD) finger in NuA4 subunits. We obtained strong evidence that the HAT activities of NuA4 and SAGA cooperate to enhance co-transcriptional recruitment of the nucleosome remodeling complex RSC, promote histone eviction from transcribed coding sequences, and stimulate the rate of Pol II elongation in vivo. We also recently extended the two-stage recruitment mechanism, via Ser5P and methylated histones, that we described for NuA4 to include the histone de-acetylase (HDA) complex Rpd3C(S). While it was known that H3 methylation by Set1 is required for de-acetylation of nucleosomes by Rpd3C(S), we showed that Rpd3C(S) is recruited in the absence of Set1, but in a manner stimulated by Cdk7/Kin28, and that a reconstituted Rpd3C(S) complex binds specifically to Ser5P-CTD synthetic peptides in vitro. We further demonstrated that the HDAs Rpd3, Hos2, and Hda1 have overlapping functions in de-acetylating coding-sequence nucleosomes and observed a strong correlation between increased acetylation and lower histone occupancy in single, double, and triple HDA mutants. These results strongly support our conclusion that histone acetylation is a key determinant of transcription-coupled nucleosome disassembly in coding sequences.
Pol II CTD kinases Bur1 and Kin28 promote an Spt5-independent pathway for recruitment of transcription elongation factor Paf1C.
Previously, we showed that the cyclin-dependent kinase (Cdk) Bur1 is recruited to promoters through its direct interaction with the Ser5-phosphorylated Pol II CTD (generated by Kin28), phosphorylates Ser2 in CTD repeats near the promoter, and stimulates Ser2-CTD phosphorylation at promoter-distal sites by the Cdk Ctk1. Recently, it was demonstrated that Bur1 also phosphorylates C-terminal repeats (CTRs) of elongation factor Spt5 in a manner that facilitates co-transcriptional recruitment of the elongation factor Paf1 complex (Paf1C). However, the biochemical function of the phosphorylated Spt5 CTR in Paf1C recruitment was unknown. It was also unknown whether the phosphorylated Pol II CTD participates in Paf1C recruitment. Our recent studies indicate that native Paf1C and Paf1C subunit Cdc73 bind specifically to both phosphorylated Spt5 CTRs and Ser2,Ser5-diphosphorylated Pol II CTD repeats in vitro and that mutations eliminating these binding activities of Cdc73 reduce Paf1C recruitment in vivo. To establish that Kin28 and Bur1 promote Paf1C independently of Spt5, we generated phosphomimetic (aspartate) substitutions in the Spt5 CTR and showed that they can sustain Paf1C recruitment in otherwise wild-type cells, but not following inactivation of an analog-sensitive variant of Bur1 in vivo. Inhibiting Kin28 reduces phosphorylation of both Spt5 and Ser2 of the CTD, consistent with Kin28 stimulation of Bur1 recruitment; however, inactivation of Kin28 also impairs Paf1C recruitment in the presence of the Spt5 phosphomimetic CTR. These results identify an Spt5-independent pathway of Paf1C recruitment and suggest that Ser2,Ser5-diphosphorylated CTD repeats and the Spt5 phospho-CTR mediate parallel pathways of Paf1C recruitment that both depend on Kin28 and Bur1.
Evidence that phosphatidylinositol (PI) 3-kinase Vps34 acts in the nucleus to promote transcription elongation by Pol II
Previously, we reported that disruption of vesicular protein trafficking at the late endosome/mulitvesicular body (MVB) in a subset of vps mutants impedes the ability of nucleus-localized and UAS-bound Gcn4 to activate transcription. Noting previous reports linking certain Vps factors that function at the MVB (the ESCRT-II complex) to transcription elongation and the co-localization of PI 3-kinase Vps34 with nuclear sites of transcription in plants, we recently explored whether yeast Vps factors might participate in transcription directly in the nucleus. Remarkably, our chromatin immunoprecipitation (ChIP) analysis revealed that Vps34 and Vps15 (a protein kinase that activates Vps34) are associated with transcribed coding sequences in a manner dependent on transcriptional activation by Gcn4 or Gal4 (at their cognate target genes), a manner dependent on the TATA element and PIC assembly at the Gcn4 target ARG1, and in a manner stimulated by Pol II CTD kinase Kin28—all features characteristic of Pol II elongation factors. Indeed, vps15 and vps34 mutants display several phenotypes of elongation factor mutants, including sensitivity to 6-azauracil (6-AU) and mycophenolic acid, defective IMD2 induction by 6-AU, and reduced mRNA production from long, GC-rich transcription units (e.g., bacterial lacZ) driven by the GAL1 promoter relative to native GAL1. Importantly, we also demonstrated a reduced rate of Pol II run-off from a PGAL1-PHO5::lacZ reporter after promoter shut-off in vivo. Consistent with a role for Vps15/Vps34 in stimulating Pol II elongation, we found that vps15 and vps34 mutations are synthetically "sick"—with mutations affecting elongation factor complexes DSIF (spt4) and THO (thp1). The results suggest an unexpected second function for Vps15/Vps34 in stimulating transcription elongation in the nucleus.
Mechanism and regulation of the GEF function of translation initiation factor eIF2B
In translation initiation, the Met-tRNAi is recruited to the 40S subunit in the TC with GTP-bound eIF2, and AUG recognition evokes hydrolysis of the GTP, releasing eIF2-GDP from the ribosome and Met-tRNAi into the P site. The eIF2-GDP is recycled to eIF2-GTP by the guanine nucleotide exchange factor (GEF) eIF2B to prepare for the next round of translation initiation. The GEF function of eIF2B is down-regulated in amino acid–starved cells by phosphorylation of the α-subunit of eIF2 by protein kinase Gcn2, provoking a reduction in general protein synthesis and the specific translational induction of GCN4 mRNA. The eIF2B contains five subunits, but the e/Gcd6 subunit is sufficient for GEF activity in vitro. The d/Gcd2, b/Gcd7, and a/Gcn3 subunits are related in sequence and constitute a regulatory subcomplex in eIF2B that mediates tight binding of phosphorylated eIF2(aP)-GDP via the "S1 domain" in eIF2α, which is required to inhibit GEF function. Down-regulation of eIF2B by eIF2(aP) and attendant induction of GCN4 mRNA translation is not essential in nutrient-replete cells, but the eIF2B regulatory subunits d/Gcd2 and b/Gcd7 are required for cell viability under all conditions, and their indispensable functions are not well understood. We showed recently that (i) depletion of b/Gcd7 from cells, (ii) three lethal b/Gcd7 amino acid substitutions, and (iii) a synthetically lethal combination of substitutions in b/Gcd7 and eIF2α all impaired eIF2 binding to eIF2B, without reducing e/Gcd6 abundance in the eIF2B complex. These and other findings provide strong evidence that, beyond its regulatory role in mediating eIF2B inhibition by phosphorylated eIF2, b/Gcd7 is essential for binding to the eIF2B substrate (unphosphorylated eIF2-GDP).
Roles of eIF4G in mRNA activation and determining translational efficiencies in vivo

Click image to enlarge.
Figure 1. Closed-loop formation via cap-poly(A) interaction mediated by eIF4G and PABP is not critical for translation in yeast cells.
A. Binding of eIF4E to the cap structure of mRNA and poly(A) binding protein (PABP) to the poly(A) tail, together with binding of eIF4E and PABP to their adjacent binding domains in eIF4G, enables formation of the closed-loop mRNP with the cap and poly(A) tail juxtaposed. It is widely held that this intermediate is crucial for attachment of the 43S pre-initiation complex (PIC) near the mRNA cap structure to initiate scanning and selection of the AUG start codon. The 43S PIC consists of the small 40S subunit loaded with initiation factors (eIFs) 3, 1, 5, and 1A and the TC comprised of eIF2-GTP-Met-tRNAi. The GTP is symbolized by a green diamond and the tRNAi as a fork.
B. We showed that elimination of the PABP-binding domain in eIF4G has no effect on translation initiation in yeast cells as long as the RNA-binding domain at the N-terminus of eIF4G (RNA1) is intact. This finding indicates that formation of the closed loop mRNP via cap-poly(A) interaction is dispensable in vivo. We proposed that PABP-eIF4G interaction is only one of several redundant interactions, including RNA1:mRNA association and eIF4E-cap interaction, that stabilize eIF4G binding to mRNA, which is likely to be the key event for 43S PIC attachment rather than circularization per se.
Attachment of the 43S PIC near the 5′ end of mRNA is a key step in the translation initiation pathway that is enhanced by (i) binding of eIF4F (eIF4E, eIF4G, eIF4A) to the cap structure at the 5′ end of mRNA, (ii) poly-A–binding protein (PABP) binding to the mRNA poly(A) tail, and (iii) PABP–eIF4G interaction. Together, these interactions produce a "closed-loop" mRNP thought to be crucial for 43S PIC attachment (Figure 1A). Budding yeast contains two functionally interchangeable eIF4G isoforms (encoded by TIF4631 and TIF4632). Key evidence that PABP–eIF4G interaction is important in vivo originally came from the finding that, in yeast cells lacking eIF4G2, a temperature-sensitive mutation in the eIF4E-binding site of eIF4G1 (tif4631–459) is synthetically lethal with an N-terminal truncation that eliminates the PABP–binding domain of eIF4G1 (-DN300). However, we found that an internal deletion of the PABP–binding domain of tif4631–459 is not lethal and that, to produce a lethal reduction in eIF4G1–459 function, the RNA-binding domain at the extreme N-terminus ("RNA1") must be removed simultaneously. We further showed that the region between RNA1 and the PABP binding domain contains two segments conserved in yeast eIF4G homologs—"Box1" and "Box2"—that also functionally overlap with the PABP-binding domain in vivo. We obtained biochemical evidence that RNA1, Box1, Box2, and the PABP–binding domain all contribute to assembly of native eIF4G∙mRNA∙PABP complexes, quantified by assaying mRNA-dependent (RNAse–sensitive) co-immunoprecipitation of PABP with eIF4G from cell extracts. In collaboration with Jon Lorsch's group, we found that RNA1 and Box1 both enhance direct PABP binding to the eIF4G1 N-terminal domain (NTD), and that Box1 contributes to RNA binding by the eIF4G1 NTD in vitro. Thus, while the eIF4G–PABP interaction is important, RNA binding by the eIF4G1 NTD makes an overlapping contribution to mRNA activation in vivo, and several segments in the NTD contribute to the PABP– and RNA–binding activities of the eIF4G1 NTD. Our results are significant in demonstrating that closed-loop mRNP formation via cap-poly(A) interaction—mediated by PABP–eIF4G interaction—is nonessential in vivo (Figure 1B). We propose that the eIF4G–PABP interaction represents only one of several interactions that stabilize eIF4G binding to mRNA, which is likely to be the critical event in promoting 43S attachment.
In addition to eIF4F's role in promoting 43S attachment to mRNA, the RNA helicase activity of eIF4A likely facilitates scanning through the secondary structure in the 5′ UTR, and eIF4F is expected to be critical in determining translational efficiencies (TEs) among different mRNA. We tested this hypothesis by measuring the effect of depleting eIF4G in vivo on TEs genome-wide, using DNA microarrays to quantify the ratio of mRNA abundance in polysomes versus total RNA. Both eIF4G isoforms were eliminated in a strain lacking TIF4632 and harboring a tif4631–td degron allele, permitting transcriptional repression and rapid degradation of eIF4G1 in non-permissive conditions. The elimination of eIF4G reduced protein synthesis rates about three-fold and arrested cell growth but, surprisingly, the TEs of most mRNAs were not greatly affected. An intriguing consequence of eIF4G depletion, however, was to narrow the range of TE values by reducing translation of many mRNAs that exhibit higher-than-average TEs in wild type while increasing translation of other mRNAs with lower-than-average TE values. Thus, while eIF4G is not essential for translation of any mRNAs in yeast, it is an important rate-enhancing factor and promotes the differentiation of TEs among mRNAs to an extent that is essential for cell division.
An upstream ORF with non–AUG start codon is translated in vivo but dispensable for translational control of GCN4 mRNA.
A recent genome-wide analysis of ribosome locations in mRNAs of Saccharomyces cerevisiae revealed an unexpectedly high level of translation of upstream open reading frames (uORFs) that initiate with non–AUG, near-cognate start codons in many different transcripts. Two such non-AUG–initiated uORFs (nAuORFs 1 and 2) occur in GCN4 mRNA upstream of the four AUG–initiated uORFs (uORFs 1–4) that are known to mediate increased translation of GCN4 mRNA in response to amino acid starvation and the attendant phosphorylation of eIF2a by Gcn2. We tested the proposal that translation of these nAuORFs plays an important role in translational control of GCN4 mRNA. We verified that nAuORF2 is translated in vivo by demonstrating β-galactosidase production from lacZ coding sequences fused to nAuORF2 in a manner abolished by replacing its AUA start codon with the non-cognate triplet AAA; however, translation of nAuORF1 was not detected by the same approach. Importantly, replacing the near-cognate start codons of both nAuORFs with non-cognate triplets had little or no effect on either the repression of GCN4 translation in non-starved cells or its derepression in response to histidine limitation, nutritional shift-down, or treatment with rapamycin, hydrogen peroxide, or methyl methanesulfonate. In addition, we found no evidence that initiation from the AUA codon of nAuORF2 is substantially elevated or dependent on Gcn2, the sole eIF2a kinase of yeast, in histidine-deprived cells. Thus, although nAuORF2 is translated in vivo, it appears that this event is not stimulated by eIF2a phosphorylation and does not significantly influence GCN4 translational induction under a variety of starvation or stress conditions.
Additional Funding
- International Human Frontiers of Science Program Grant RGP0028
Publications
- Ginsburg DS, Govind CK, Hinnebusch AG. NuA4 lysine acetyltransferase Esa1 is targeted to coding regions and stimulates transcription elongation with Gcn5. Mol Cell Biol. 2009; 24:6473-6487.
- Chiu WL, Wagner S, Herrmannová A, Burela L, Zhang F, Saini AK, Valasek L, Hinnebusch AG. The C-terminal region of eIF3a promotes mRNA recruitment, scanning and, together with eIF3j and the eIF3b RRM, selection of AUG start codons. Mol Cell Biol. 2010; 30:4415-4434.
- Jedidi I, Zhang F, Qiu H, Stahl SJ, Palmer I, Kaufman JD, Nadaud PS, Mukherjee S, Wingfield PT, Jaroniec CP, Hinnebusch AG. Activator Gcn4 employs multiple segments of Med15/Gal11, including the KIX domain, to recruit Mediator to target genes in vivo. J Biol Chem. 2010; 285:2438-2455.
- Saini AK, Nanda JS, Lorsch JR, Hinnebusch AG. Regulatory elements in eIF1A control the fidelity of start codon selection by modulating tRNAiMet binding to the ribosome. Genes Dev. 2010; 24::97-110.
- Govind CK, Qiu H, Ginsburg DS, Ruan C, Hofmeyer K, Hu C, Swaminathan V, Workman JL, Li B, Hinnebusch AG. Phosphorylated Pol II CTD recruits multiple HDACs, including Rpd3C(S), for methylation-dependent deacetylation of ORF nucleosomes. Mol Cell. 2010; 39:234-246.
Collaborators
- Jo Lorsch, PhD, The Johns Hopkins University School of Medicine, Baltimore, MD
- Leos Valášek, PhD, Institute of Microbiology, Academy of Sciences of the Czech Republic, Prague, Czech Republic
- Paul Wingfield, PhD, National Institute of Arthritis and Musculoskeletal and Skin Diseases, NIH, Bethesda, MD
- Christopher Jaroniec, PhD, Ohio State University, Columbus, OH
- Bing Li, PhD, University of Texas Southwestern Medical Center at Dallas, Dallas, TX
- Jerry Workman, PhD, The Stowers Institute for Medical Research, Kansas City, MO
Contact
For more information, email hinnebua@mail.nih.gov or visit sncge.nichd.nih.gov.