You are here: Home > Unit on Cellular Communication
Mechanisms of Cellular Communication during Development
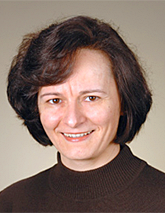
- Mihaela Serpe, PhD, Head, Unit on Cellular Communication
- Young-Jun Kim, PhD, Visiting Fellow
- Carolyn E. Peluso, PhD, Postdoctoral Fellow
- Peter Nguyen, Biological Laboratory Technician
- Liana Bonanno, BS, Postbaccalaureate Fellow
The long-term goal of our unit is to elucidate molecular mechanisms that regulate cell-cell communication during development. We are interested in two related key questions in cellular communication: (i) how tissues are patterned and correctly connected by short- and long-range signals and (ii) how cells' structures and functions are coordinated at short range with those of their neighbors. We study these processes by focusing on early developmental patterning and the development of a specialized cell-cell interaction zone—the neuromuscular junction (NMJ).
We are particularly interested in signaling of growth and differentiation factors by TGF-beta, one of the largest families of signaling molecules. Highly conserved throughout the animal kingdom, TGF-beta signaling factors (such as bone-morphogenic proteins and activins) have the ability to function as morphogens, that is, to specify cell fate in a concentration-dependent manner. In addition, these signaling factors provide a mechanism for coupling a cell to its neighbors and to its environment, so that the cell has the necessary plasticity to respond appropriately to changes around it, in its environment, or even to its own state. We use the Drosophila melanogaster model system and a variety of molecular and biochemical approaches to study genes that modulate the function of TGF-beta–type signals.
Shaping BMP morphogen gradients through enzyme-substrate interactions
Spatially non-uniform distributions of secreted morphogens guide tissue development in a highly reproducible and robust manner. In early embryonic patterning of insects and chordates, BMPs (bon-morphogenic proteins) guide dorsal/ventral patterning through a complex network that relies on the spatial distribution of a BMP ligand (Dpp, BMP2/4) and a BMP antagonist (Sog/Chordin). The regulation of Sog in Drosophila and Chordin in vertebrates has, however, acquired distinct characteristics. We use biochemical, genetic, quantitative image analysis, and computational modeling studies to explore the molecular etiology of these differences.
Formation of the Dpp activity gradient requires several secreted modulators. In the Drosophila early embryo, Dpp is bound in a complex containing Short gastrulation (Sog). The complex inhibits binding of Dpp to its receptors in lateral regions and facilitates long-range ligand diffusion, shuttling Dpp from the lateral domain toward the midline. A key component that helps create flux is the cleavage of Sog by Tolloid (Tld), a metalloprotease of the BMP-1 family. The net movement of Dpp dorsally is generated by reiterated cycles of complex formation, diffusion, and destruction by Tld. Sog plays both positive and negative roles in regulating BMP activity, a phenomenon previously referred to as the "Sog paradox." The negative role comes from blocking access of ligands to receptors; the positive effect comes from Sog's ability to facilitate Dpp diffusion.
Although Chordin is thought to be the functional homolog of Sog, it acts only as an inhibitor and cannot promote long-range Dpp signaling when introduced into Drosophila. At the molecular level, Sog and Chordin differ in that cleavage of Sog by Tld requires the BMP ligand as an obligatory co-substrate while Chordin does not. We proposed that the dependence of Sog's destruction on BMP makes Sog a more efficient BMP transporter and results in long-range BMP signaling. To test this hypothesis, we first characterized the Sog sites processed by Tld and derived a consensus cleavage sequence. The requirement for the obligatory co-substrate for Sog cleavage is thought to indicate a BMP–induced conformational modification that allows the Sog–BMP complex, but not Sog alone, to fit into the catalytic pocket of the enzyme. We modeled the Tld catalytic domain by using the crystal structures available for related enzymes and studied the enzyme-substrate interactions between Tld and either Sog or Chordin. We found that several residues are responsible for rendering Sog (but not Chordin) destruction dependent on BMP binding. Changing corresponding residues at the cleavage site indeed altered the co-substrate requirements. The strongest effect was for a Sog variant in which both sites 1 and 2 were rendered BMP–independent for processing, designated Sog-i for "independent of BMP for cleavage." At the sequence level, Sog-i is markedly different from Chordin but resembles Chordin in how it is processed by Tld: Sog-i exhibits significant BMP–independent processing by Tld, which is enhanced in the presence of BMP ligands. These changes do not affect Sog's ability to bind to BMPs and/or inhibit their signaling.
To test the biological effect of the Sog variants on the BMP–morphogen gradient profile, we constructed transgenic fly lines that permit normal spatial and temporal expression of Sog proteins at endogenous levels, under the control of the lateral stripe enhancer from the sog promoter. The lines rescued sog mutants and trans-heterozygous combinations (sog−/−) to viable and fertile adults. We examined the profile of the BMP–morphogen gradients in stage 5 embryos by following the accumulation of activated/phosphorylated Mad (P-Mad), the effector of the BMP signaling pathway. In embryos in which Sog-i replaced the endogenous Sog, the boundaries of the P-Mad–positive domains were more diffuse, with shallower slopes evident in the cross-section profile. In collaboration with David Umulis, we analyzed and quantified the differences in BMP signaling profiles by measuring the spatial derivative of P-Mad in the cross-section. The slopes for 2x sog-i embryos were noticeably shallower than for wild type (wt) but did not correspond to the lower total amount of Sog protein, i.e., sog+/− embryos. The biological consequences of replacing Sog with Sog-i could not be explained simply by differences in Sog protein levels between the sog-wt and sog-i transgenes. The P-Mad–positive domains in embryos with four copies of sog-i remained wide, the boundaries were diffuse, and the slopes of the cross-section profiles were shallower. Such changes in the gradient profiles had a major impact on Drosophila patterning by affecting cell-fate allocation and tissue size. For example, the sharp and narrow BMP signaling domain in wt embryos induced formation of an amnioserosa field of approximately 200 cells in stage-13 embryos. Replacement of endogenous sog with sog-i produced a statistically significant increase in the amnioserosa field (about 300 cells).
Moreover, our comparison between sog-wt and sog-i embryos suggests that BMP–dependent Sog processing reduces embryo-to-embryo variability in P-Mad levels and amnioserosa fields. When we calculated the coefficient of variation (standard deviation/width) within each genotype, we found that embryos with two or four sog-wt copies showed less variability in signaling width and amnioserosa fields than their sog-i counterparts. Our results indicate that a ''Chordin-like'' Sog is less able to reliably support patterning of the early Drosophila embryo. Thus, the acquisition of BMP–dependent Sog processing during evolution facilitated long-range ligand diffusion and formation of the robust, bi-stable BMP–morphogen gradients required for early Drosophila patterning.
Drosophila Neto essential for clustering glutamate receptors at the neuromuscular junction
Ionotropic glutamate receptors (iGluRs) are a critical component of the vertebrate central nervous system and mediate the majority of rapid excitatory neurotransmission. The Drosophila neuromuscular junction (NMJ) is a glutamatergic synapse, similar in structure and physiology to mammalian central AMPA/kainate synapses. In flies, each NMJ is unique and identifiable, and synapses are large and accessible for electrophysiological and optical analysis, making the Drosophila NMJ a highly suitable genetic system for the study of synapse development. The subunits that form the iGluRs are known and relatively well studied. However the mechanisms that control iGluR clustering and stabilization at the postsynaptic densities (PSDs), a key step that confers functionality to the nascent synapse, remain a mystery. Several mechanisms have been identified that regulate the subunit compositions and the extent of iGluR synaptic localization, but no molecules other than the receptors themselves have been shown to be absolutely required for clustering of the receptor complexes.
One possible link in understanding the trafficking and clustering of iGluRs at the fly NMJ could be provided by the emerging families of auxiliary subunits. Recent discoveries in vertebrates revealed the extraordinary diversity and richness that auxiliary subunits impart to vertebrate iGluR function. Auxiliary subunits are transmembrane proteins that avidly and selectively bind to mature iGluRs and form stable complexes at the cell surface. They can modulate the functional characteristics of iGluRs and may mediate surface trafficking and/or targeting to specific subcellular compartments. It has been reported that several Drosophila genes encode auxiliary subunits, but none have yet been characterized.
We characterized a novel, essential locus—neto (neuropillin and tolloid-like)—required for iGluR clustering at the Drosophila NMJ. Drosophila Neto has two vertebrate homologs called Neto-1 and Neto-2 that were recently shown to modulate the pharmacological properties of selective kainate-type receptors. Neto1/Neto2 double knockout mice have defects in long-term potentiation and learning and memory but are nevertheless viable. In contrast, the Drosophila neto mutants we generated are homozygous lethal; they die as paralyzed embryos and lack any body wall peristalsis and hatching movements. Muscle expression of Neto rescued the paralyzed neto-null embryos to viable, fertile adults, indicating that Neto activity is essential in the striated muscle.
Neto is present at two distinct locations in the striated muscle on the muscle surface: in a striped pattern reminiscent of the T-tubules and concentrated at the NMJ. At the NMJ, Neto co-localizes with iGluRs in puncta juxtaposed to sites of neurotransmitter/glutamate release, the active zones. The iGluRs of the Drosophila NMJ are hetero-tetrameric complexes composed of the three essential subunits—IIC, IID, IIE, and either IIA or IIB. Embryos lacking any of the essential subunits, or without IIA and IIB together, are paralyzed and cannot hatch into the larval stages. The essential subunits are required not only for viability but also for the clustering of the other iGluRs at the NMJ. The similarities in subcellular localization and loss-of-function phenotype of Neto and iGluRs raised the possibility that Neto may be important for iGluRs clustering at the NMJ.
Our histological and physiological studies revealed that Neto is required for clustering of iGluRs at the onset of synaptogenesis as well as during later developmental stages. iGluRs begin to concentrate at the synaptic cleft soon after contact between the motoneuron growth cone and muscle. In neto-null embryos, however, there are significantly fewer clusters and they are smaller and remain relatively far from the presynaptic arbors. The defects were not caused by inappropriate axon guidance or lack of adhesion, given that the pre- and postsynaptic partners appeared properly aligned, and the pre-patterning step seemed normal. However, iGluRs do not cluster in the absence of Neto and remain scattered in small, extra-synaptic aggregates. The lethality and clustering defects in neto mutants are completely rescued when Neto activity is provided in the striated muscle.
To understand how Neto controls the clustering of iGluRs, we explored the timing of Neto clustering at the NMJ by using live imaging of embryos with muscle-expressed Neto-eGFP. Neto accumulates and clusters at the NMJ as early as 14 hours after egg laying, when the iGluRs begin to accumulate and cluster at the synapse. Most important, Neto-positive puncta could no longer be detected in gluRIID−/−-null embryos. Thus, Neto and iGluRs depend on each other for clustering at the NMJ. Simply stated, Neto functions as a non-channel, essential subunit of the iGluR complexes.
Our data fit best with the model in which Neto and the iGluRs are engaged in targeting each other to the PSDs via a direct interaction. Neto likely binds to iGluR complexes on the surface of muscle followed by trafficking to the synaptic junction. Our model is consistent with the Neto/iGluRs co-dependence for clustering at the synapse that we described: only components engaged in a productive complex could traffic and be stabilized at the NMJ. The model also predicts that, at suboptimal Neto levels, iGluRs would accumulate on the muscle surface at extra-junctional locations. Indeed, such appears to be the case because, in neto hypomorphs, we detected GluRIIA on the muscle surface, accessible by antibodies in the absence of detergents. In addtion, overexpression of Neto in the muscle increased evels of iGluRs at extra-junctional locations and reduced NMJ growth.
An intriguing question is why the requirements for Neto differ dramatically among various organisms. Neto1/Neto2 double-knockout mice have defects in long-term potentiation and learning and memory but are viable. More important, Neto1 and Neto2 are not essential for iGluRs clustering. In contrast, Drosophila neto-null mutants are embryonic lethal, and Neto is absolutely required for iGluRs clustering. It is tempting to speculate that Neto might have attained tissue- or context-specific roles in the modulation of iGluRs. Thus, Netos constitute a family of proteins conserved from flies to humans that influence the function of glutamatergic synapses and have acquired species- and tissue-specific roles during evolution.
Publications
- Peluso CE, Umulis D, Kim YJ, O'Connor MB, Serpe M. Shaping BMP morphogen gradients through enzyme-substrate interactions. Dev Cell 2011;21:375-383.
Collaborators
- Seth S. Blair, PhD, University of Wisconsin, Madison, WI
- Chi-Hon Lee, PhD, Program on Cellular Regulation and Metabolism, NICHD, Bethesda, MD
- Mark Mayer, PhD, Program in Developmental Neuroscience, NICHD, Bethesda, MD
- Michael B. O’Connor, PhD, University of Minnesota, Minneapolis, MN
- David Umulis, PhD, Purdue University, West Lafayette, IN
- Bing Zhang, PhD, University of Oklahoma, Norman, OK
Contact
For more information, email serpemih@mail.nih.gov or visit ucc.nichd.nih.gov.