You are here: Home > Section on Cell Biophysics
Cell Biophysics
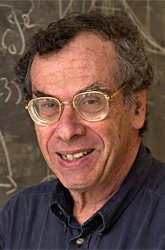
- Ralph Nossal, PhD, Head, Section on Cell Biophysics
- Adrian Begaye, BA, BS, Postbaccalaureate Fellow
- Hacène Boukari, PhD, Guest Researcher
- Jennifer Galanis, MD, Guest Researcher
- Dan Sackett, PhD, Staff Scientist
We study elements of cell processes involved in signal transduction, protein trafficking, microtubule biology, and cell division. We focus on the origination and transformations of supramolecular cellular assemblages such as protein-coated endocytic vesicles, metabolic signaling complexes, and cytoskeletal structures. In our research, we develop and apply novel methodologies based on mathematical and physical principles. For example, we have constructed specialized fluorescence-based optical instrumentation to study the dynamics of supramolecular processes, and used advanced electromagnetic scattering techniques to examine structures on nanoscopic length scales. We are particularly interested in the way cellular activities are coordinated in space and time.
Biophysical methods and models
We develop new analytical methods to study biological structure and behavior. These include physical methods based on fluorescence correlation spectroscopy (FCS), quantitative microscopy, and Fourier transform analysis. In particular, we devise techniques that permit us to examine the motion of particles within concentrated polymer solutions and dense, interconnected polymer matrices. To do so, we often employ models of non-biological origin to establish the physical basis of the methods. Recently, we focused on discerning how the swelling or shrinking of a cross-linked hydrogel affects the movement of embedded nanoparticles (Boukari et al., 2008). We have employed related techniques to examine the translocation of viruses through vaginal secretions, our goal being to understand how HIV and other viruses involved in sexually transmitted diseases penetrate cervical mucus and other protective barriers to reach the cells they infect. Particle-tracking studies of individual virions indicate that most virus particles slow down more than 100-fold when compared with their movement in water and that typical diffusion does not drive the virions’ translocation. Rather, a major factor is relaxation of the polymer network of the mucus following mechanical perturbation of a sample, giving rise to a combination of anomalous diffusion and occasional jumps dependent upon the microenvironments of individual virus particles (Boukari et al., 2009).
The above phenomena occur in relatively dense media, in which significant light scattering from the surrounding matrix might cause problems. In FCS measurements, for example, analysis requires reliable determination of the illuminated volume, but the incident beam may be distorted by multiple scattering. Hence, we have carried out Monte Carlo simulations to examine the effects of scattering induced by a crowded solution of spherical nanoparticles, finding that, as the concentration or size of the particles increases, the beam-spot broadens along the axial plane. Also, the incident intensity at the focal plane decreases. Depending on the scattering properties of the medium, one may need to be account for such effects when using FCS to investigate optically dense tissue.
Clathrin lattice formation and supramolecular assembly
Receptor-mediated endocytosis involves the formation of vesicles surrounded by closed polyhedral, cage-like structures assembled from a three-legged heteropolymer composed of three clathrin heavy/light chain complexes joined at a common hub (the "clathrin triskelion"). In devising physical theories to understand how such clathrin-coated vesicles (CCVs) arise, we have developed various new quantitative methods of physical analysis. Most recently, we used novel computer-based structural modeling, combined with dynamic light scattering (DLS), static light scattering (SLS), and small angle neutron scattering (SANS), to examine conformations of clathrin triskelia in solution. We were interested in determining if and how clathrin triskelia change their shape when they leave solution to assemble into coat-associated cages. We showed that triskelia are puckered when free in solution, although they exhibit a somewhat different conformation than when incorporated into a reconstituted clathrin basket, suggesting that the mechanical properties of triskelia must be taken into account. Therefore, we developed a novel scheme, based on SANS, to assess the flexibility of the triskelia, permitting us to determine quantities that previously could be deduced only by somewhat indirect methodology. Results substantiate our earlier inferences that triskelia are semiflexible polymeric structures capable of bending when integrated into polyhedral coats of various sizes and shapes (Ferguson et al.). Moreover, using atomic force microscopy (AFM), we were recently able to obtain time sequence images of individual, wet triskelia resting on mica surfaces. These records demonstrate conformational fluctuations of the individual triskelial legs. Other AFM studies of dried samples yielded images with resolution comparable to that obtainable by electron microscopy. Increased numbers of triskelion dimers and assembly intermediates, as well as structures with dimensions similar to those of clathrin cages, were observed when triskelia were immersed in a low salt, low pH buffer. We are now applying the knowledge gained from these and related studies to investigate clathrin pit formation at the plasma membrane, focusing on discovering how the binding of clathrin to accessory proteins such as AP-2 and AP-180 influences clathrin coat nucleation.
In a related study, undertaken to gain deeper knowledge of the effects of molecular crowders on supramolecular assembly, we employed a biomimetic model consisting of macroscopic rods immersed in a fluid of small spheres. Mechanical shaking played a role analogous to thermal excitation. We found that, depending on the number densities of the constituents, the rods self-assemble into linear polymer-like structures when confined to quasi two-dimensional spaces. These structures are also predicted by equilibrium simulations, suggesting that entropy maximization is the driving force for bundling. Such results may provide insight into the role of seemingly extraneous solution constituents in the assembly of oligomeric structures at interfaces.
Complex systems biophysics
We continue to use advanced physical and mathematical methods to understand the biophysics of complex cellular activities. The role of phosphoinositide metabolism in the biogenesis of vesicles involved in cellular transport events is one such process. We recently formulated a mathematical model to investigate the involvement of 3′ phosphoinositides in the biogenesis of clathrin-coated and other endocytic vesicles. This description of receptor-mediated endocytosis encompasses cargo recognition, phosphoinositide metabolism, and clathrin coat formation and dissolution. Our analysis, which demonstrates how interrelated kinetic elements of these processes determine whether an endocytic vesicle will form, will permit us to explain how vesicle biogenesis at specific sites is triggered by the binding of ligands to receptors and subsequent recruitment of clathrin-associated proteins. We are also interested in rationalizing the observed stochastic quality of cell response in the presence of a stimulus.
Tubulin polymers and cytoskeletal organization
We study both fundamental and applied aspects of tubulin biology. Tubulin polymers are central to various critical cell functions, including mitosis, intracellular transport, maintenance of cell morphology, cell motility, and perhaps mitochondrial function. We have devoted considerable effort to determining the ability of small molecules to alter the formation of microtubules (MT), which are the most rigid of the cytoskeletal polymers and are thus central to establishing non-spherical cell morphology. In addition, MTs have intrinsic polarity, and their cytoplasmic array provides the substrate for directional intracellular movement. Consequently, small molecules that alter MT integrity and/or dynamics can alter the physical properties of the cytoplasm. We have been studying antimitotic peptides, often of marine origin, because they are among the most potent anti–MT agents known. We have also enhanced our work with stathmin, an intracellular inhibitor of microtubule polymerization that is increased in many cancers, and we continue our interest in microtubule-stabilizing drugs.
Recently, we identified a number of new modified peptides based on the natural product tubulysin (Balasubramanian et al.). We are systematically establishing the structure-activity relationship among structural modifications of the peptide, initially focusing on mammalian cells, but later extending this analysis to protozoal parasites, using Leishmania as a model. We now have defined structural modifications that alter the cytotoxicity to mammalian cells by more than 10,000-fold, from low nanomolar concentrations to more than 10 micromolar.
We previously demonstrated that nitrosoureas inhibit migration of cancer cells by interfering with stathmin-tubulin interactions, resulting in increased survival of animals with glioblastoma. Nitrosoureas are old and established chemotherapy agents that have a number of unfortunate side effects. In an effort to discover a better compound to target stathmin, we developed a fluorescence polarization–based assay for stathmin-tubulin binding and plan to use this assay to screen libraries of potential drugs. We also continued our studies of microtubule stabilizing molecules. Our mass spectrometry–based measurements of microtubules induced by the drug peloruside contributed to a modified model of microtubule assembly. We also designed, produced, and tested an analog of the clinical drug epothilone, with the aim of increasing stability while preserving activity.
Finally, we have been studying the interactions between tubulin and supramolecular entities that affect metabolic activities of mitochondria—organelles that move along microtubules in mammalian cells. We examined the effect of tubulin binding on the function of the major mitochondrial outer membrane (MOM) channel protein VDAC (voltage-dependent anion channel) and found that nanomolar concentrations of tubulin cause closure of the channel (Rostovtseva et al.). This closure, which requires the C-terminal tail peptides found on alpha and beta tubulin, is expected to restrict exchange of ADP and ATP across the MOM. Using isolated intact mitochondria, we confirmed this effect. Added tubulin reduces the oxygen uptake associated with added ADP, as reflected in a nearly 100-fold increase in KM for ADP. Our results show that tubulin can be involved in regulating respiration in mammalian cells by interacting with VDAC on the outer membrane of mitochondria.
Publications
- Boukari H, Silva CS, Nossal R, Horkay F. Monitoring nanoprobe diffusion in osmotically-stressed hydrogels. Mater Res Soc Symp Proc 2008 1060E:LL07-13.
- Boukari H, Brichacek B, Stratton P, Mahoney SF, Lifson JD, Margolis L, Nossal R. Movements of HIV-virions in human cervical mucus. Biomacromolecules 2009 10:2482-2488.
- Ferguson ML, Prasad K, Boukari H, Sackett DL, Krueger S, Lafer EM, Nossal R. Clathrin triskelia show evidence of molecular flexibility. Biophys J 2008 95:1945-1955.
- Balasubramanian R, Raghavan B, Begaye A, Sackett DL, Fecik RA. Total synthesis and biological evaluation of tubulysin U, tubulysin V, and their analogues. J Med Chem 2009 52:238-240.
- Rostovtseva TK, Sheldon KL, Hassanzadeh E, Monge C, Saks V, Bezrukov SM, Sackett DL. Tubulin binding blocks mitochondrial voltage-dependent anion channel and regulates respiration. Proc Natl Acad Sci USA 2008 105:18746-18751.
Collaborators
- Sergey Bezrukov, PhD, Program in Physical Biology, NICHD, Bethesda, MD
- Beda Brichacek, PhD, Program in Physical Biology, NICHD, Bethesda, MD
- Robert A. Fecik, PhD, University of Minnesota, Minneapolis, MN
- Matthew Ferguson, PhD, Centre de Biochimie Structurale, Montpellier, France
- Tito Fojo, MD, Medical Oncology Branch, NCI, Bethesda, MD
- Amir Gandjbakhche, PhD, Program in Physical Biology, NICHD, Bethesda, MD
- Daniel Harries, PhD, Hebrew University, Jerusalem, Israel
- Ferenc Horkay, PhD, Program in Physical Biology, NICHD, Bethesda, MD
- Albert J. Jin, PhD, Division of Biomedical Engineering and Physical Science, ORS, Bethesda, MD
- Susan Krueger, PhD, Center for Neutron Research, NIST, Gaithersburg, MD
- Eileen Lafer, PhD, University of Texas Southwestern Medical Center, San Antonio, TX
Contact
For more information, email nossalr@mail.nih.gov.