You are here: Home > Section on Molecular Transport
Biophysics of Large Membrane Channels
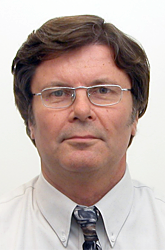
- Sergey M. Bezrukov, PhD, Head, Section on Molecular Transport
- Philip A. Gurnev, PhD, Research Fellow
- Ekaterina M. Nestorovich, PhD, Research Fellow
- Tatiana K. Rostovtseva, PhD, Staff Scientist
- Kely L. Sheldon, MS, Postbaccalaureate Fellow
Channel-facilitated transport of metabolites and other large solutes is essential to the maintenance of life. Disturbances in transport through ion channels result in a wide variety of pathologies in different organ systems, including, among others, cystic fibrosis, seizure disorders, muscular dystrophies, and cardiomyopathies. By combining physical theory with channel reconstitution experiments, this Section addresses fundamental questions of transport across cell and mitochondrial membranes. The main goal is to elucidate the physical principles and molecular mechanisms responsible for metabolite flux regulation. Our work to date has revealed previously unsuspected and surprising functional links between distinct signal transduction pathways that appear to be important during healthy development and in pathophysiology. These links open new avenues for treatment of various diseases, where regulation of transport through ion channels plays a key role.
Large ion channels are recognized as both the gateways of metabolite exchange and multifunctional membrane receptors. They are also critical components of many toxins. To study channels under precisely controlled conditions, we purify the channel-forming proteins produced by various organisms and then reconstitute them into planar lipid bilayer membranes. We explore a number of channel-forming proteins, including mammalian VDAC (Voltage-Dependent Anionic Channel from the outer membrane of mitochondria), Anthrax Protective Antigen (from Bacillus anthracis), OmpF (general bacterial porin from Escherichia coli), LamB (sugar-specific bacterial porin from Escherichia coli), alpha-Hemolysin (toxin from Staphylococcus aureus), OprF (porin from Pseudomonas aeruginosa), Alamethicin (amphiphilic peptide toxin from Trichoderma viride), and Syringomycin E (lipopeptide toxin from Pseudomonas syringae). We believe that combining work with this broad variety of channels of different origin, structure, and function in one laboratory is the most effective strategy toward elucidating major physical mechanisms of their regulation.
Physical theory of channel-facilitated metabolite transport
Our effort in physical theory concentrates on further development of the continuum diffusion model of solute dynamics in a membrane channel. The most important advance of this year was to apply our analytical approach to the so-called “entropy potentials” and to show the importance of particle-particle interactions in breaking the particle flux symmetry (Berezhkovskii et al.). Water-filled pores of biological channels usually have a complex geometry that only rarely can be approximated by a cylinder. For example, high-resolution crystallography of bacterial porins and other large channels demonstrates that their pores can be envisaged as tunnels whose cross-sections change significantly along the channel axis. For some, variation in cross-section area exceeds an order of magnitude, which leads to the so-called entropic wells and barriers in theoretical description of transport through such structures. To approach this complex problem, we analyzed transport through conical channels that is driven by the difference in particle concentrations on the two sides of the membrane. Indeed, fluxes of non-interacting particles through the same channel, inserted into the membrane in two opposite orientations, are equal because of the detailed balance. We have shown that this flux symmetry is broken by particle-particle interactions, so that one of the orientations—the orientation corresponding to the increasing channel cross section in the direction of transport—can be much more efficient under the same external conditions. Our analytical results were confirmed using three-dimensional Brownian dynamics simulations.
Physical interactions in transport regulation
This year, we were able to address a wide scope of questions ranging from van der Waals interactions of particles in liquids to electrostatic effects in channel selectivity and conductance to osmolyte-regulated hydration. One of our achievements was the elucidation of the lipid-packing–dependent partitioning of the prototypical anesthetic halothane into lipid bilayers (Weinrich et al.). Using the gramicidin A channel as a molecular probe, we found that its sensitivity to the anesthetic is highly lipid-dependent. Specifically, exposure of membranes made of lamellar dioleoylphosphatidylcholine (DOPC) to halothane in concentrations close to clinically relevant reduces channel life-times by an order of magnitude. At the same time, gramicidin channels in membranes of non-lamellar dioleoylphosphatidylethanolamine (DOPE) are little, if at all, affected by halothane. We attribute this difference in channel behavior to a difference in the stress of lipid packing into a planar lipid bilayer, wherein the higher stress of DOPE packing reduces halothane partitioning into the hydrophobic interior. Thus, our findings suggest a new role for the physical interactions originating from the stress of lipid packing, revealing a previously unknown mechanism of anesthetic efficacy regulation.
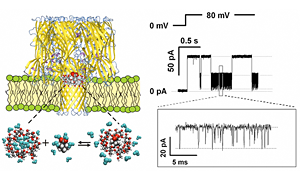
Click image to enlarge.
Figure 1. Alpha-hemolysin nanopore as a single-molecule sensor to follow the effect of different salts on the complexation reaction of gamma-cyclodextrin and adamantane carboxylate
By monitoring transient obstruction of the current through the nanopore due to binding of the cyclodextrin-adamantane complex, it is possible to study kinetics underlying the dynamic equilibrium in the presence of various cosolutes.
Another achievement was to use an ion channel nanopore as a single-molecule sensor (Figure 1) to follow the effect of various salts on adamantane-cyclodextrin complexation (Gurnev et al.). Since the pioneering experiments of Hofmeister on the “salting out” of aqueous solutions of proteins, a vast body of research has taught us that cosolutes can increase or decrease complex stability, whether preferentially excluded from or attracted to the surfaces of the associating molecules. Now studies of the underlying kinetics at a single-molecule level open the possibility of instructive inquiry into the molecular basis of the phenomenon. Surprisingly, we found that not only the stability of the complex, as measured by the life time of the adamantine–cyclodextrin complex, but also the accessibility of the complexation site can be critically increased by preferentially excluded cosolutes. The significance of these findings follows from the fact that all biochemical reactions, such as protein folding, drug binding to the target, protein–ligand and protein–DNA interactions, require release or restructuring of water layers associated with interacting molecular surfaces in the crowded environment of the cell. Therefore, understanding the mechanisms of molecular surface hydration is crucially important for resolving the fundamental questions of molecular recognition and assembly, drug design, and metabolite transport.
A new role for the "old" protein tubulin in the regulation of mitochondria respiration
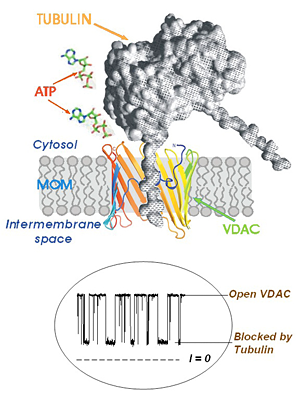
Click image to enlarge.
Figure 2. A model of tubulin-induced VDAC permeation block
One of tubulin's negatively charged C-terminal tails partially blocks the channel conductance by entering the VDAC pore in its open state and binding to the positively charged channel walls. This is seen on the traces of current through a single channel (bottom inset) as tubulin-induced fast flickering of channel conductance between open and blocked states.
Regulation of mitochondrial outer membrane (MOM) permeability has dual importance: in normal metabolite and energy exchange between mitochondria and cytoplasm and thus in control of respiration; and in apoptosis by release of apoptogenic factors into the cytosol. However, the mechanism of this regulation involving the VDAC, the major channel of the MOM, remains controversial. A long-standing puzzle is that in permeabilized cells, adenine nucleotide translocase (ANT) is less accessible to cytosolic ADP than in isolated mitochondria. We solved this puzzle by finding a missing player in the regulation of MOM permeability: the cytoskeletal protein tubulin (Rostovtseva and Bezrukov; Rostovtseva et al.). We showed that nanomolar concentrations of dimeric tubulin induce voltage-sensitive reversible closure of VDAC reconstituted into planar phospholipid membranes (Figure 2). Tubulin strikingly increases VDAC voltage sensitivity and, at physiological salt conditions, can induce VDAC closure at less than 10 mV transmembrane potentials. Experiments with isolated mitochondria confirm these findings. Tubulin added to isolated mitochondria decreases ADP availability to ANT, partially restoring the low MOM permeability found in permeabilized cells. Our findings suggest a previously unknown mechanism of regulation of mitochondrial energetics, governed by VDAC and tubulin at the mitochondria–cytosol interface. This tubulin–VDAC interaction requires the presence of tubulin anionic C-terminal tail peptides. The significance of this interaction may be reflected in the evolutionary conservation of length and anionic charge in the C-terminal tails throughout eukaryotes, despite wide changes in the exact sequence. Additionally, tubulins that have lost significant length or anionic character of C-terminal tails are only found in cells that do not have mitochondria. Thus, the discovered VDAC–tubulin interaction provides a previously absent link between Ca2+ homeostasis, cytoskeleton/microtubule activity, mitochondria, and apoptosis (Figure 3). This finding may help identify the mechanisms of mitochondria-associated action of chemotherapeutic microtubule-targeting drugs such as paclitaxel and vincristine and understand why and how cancer cells preferentially use inefficient glycolysis rather than oxidative phosphorylation (Warburg effect).
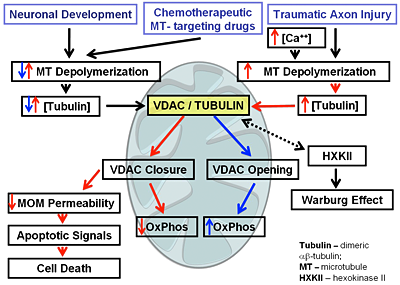
Figure 3. A diagram of immediate implications of the discovered VDAC-tubulin interaction
Additional Funding
- NIAID intramural biodefense grant (2009, ongoing) “From anthrax to botulism, cholera, and epsilon toxin: In search for broad-spectrum antitoxins that block virulent nanopores”
- USUHS CNRM grant (2009, ongoing) “Membrane biophysics of traumatic brain injury”
Publications
- Berezhkovskii AM, Pustovoit MA, Bezrukov SM. Entropic effects in channel-facilitated transport: inter-particle interactions break the flux symmetry. Phys Rev E 2009 80:020904(R).
- Weinrich M, Rostovtseva TK, Bezrukov SM. Lipid-dependent effects of halothane on gramicidin channel kinetics: a new role for lipid packing stress. Biochemistry 2009 48:5501-5503.
- Gurnev PA, Harries D, Parsegian VA, Bezrukov SM. The dynamic side of the Hofmeister effect: a single-molecule nanopore study of specific complex formation. Chem Phys Chem 2009 10:1445-1449.
- Rostovtseva TK, Bezrukov SM. VDAC regulation: role of cytosolic proteins and mitochondrial lipids. J Bioenerg Biomembr 2008 40:163-170.
- Rostovtseva TK, Sheldon K, Hassanzadeh E, Monge C, Saks V, Bezrukov SM, Sackett DL. Tubulin binding blocks mitochondrial voltage-dependent anion channel and regulates respiration. Proc Natl Acad Sci USA 2008 105:18746-18751.
Collaborators
- Alexander M. Berezhkovskii, PhD, Division of Computational Bioscience, CIT, NIH, Bethesda, MD
- Daniel Harries, PhD, Hebrew University of Jerusalem, Jerusalem, Israel
- Adrian Parsegian, PhD, Program in Physical Biology, NICHD, Bethesda, MD
- Mark A. Pustovoit, PhD, St. Petersburg Nuclear Physics Institute, Gatchina, Russia
- Dan Sackett, PhD, Program in Physical Biology, NICHD, Bethesda, MD
- Valdur Saks, PhD, Université Joseph Fourier, Grenoble, France
- Michael Weinrich, MD, National Center for Medical Rehabilitation Research, NICHD, Bethesda, MD
Contact
For more information, email bezrukov@helix.nih.gov or visit smt.nichd.nih.gov.