You are here: Home > Section on Medical Biophysics
Medical Biophysics
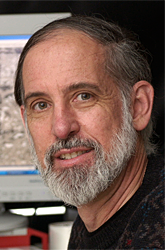
- Robert F. Bonner, PhD, Head, Section on Medical Biophysics
- Martin Ehler, PhD, Visiting Fellow
- Emily J. King, PhD, Postdoctoral Fellow
- Kimberly Tran, MS, Technical Training Fellow
- Zigurts Majumdar, PhD, Research Fellow
- Sanford Meyers, MD, Guest Researcher
- Eleanor Ory, BS, Postbaccalaureate Fellow
Currently, we are developing optical technologies to characterize early stages of disease and to monitor responses to therapy in cancer and age-related macular degeneration (AMD). We are continuing to develop our inventions of expression microdissection and laser capture microdissection for better integration with multiplex molecular analyses of specific cells and organelles extracted from complex tissue. By integrating multispectral, non-invasive, clinical retinal autofluorescence imaging with automated image analysis, we also seek to map the distribution of several intrinsic photochemicals implicated in health preservation and early disease. In pilot clinical studies, we are applying our new technology to understanding how changes in retinal spectral irradiance affect the balance between retinal photochemical pathways that, we hypothesize, drive early age-related maculopathy. Our broader goals are to quantify earlier stages of local molecular imbalance throughout the retina and to develop reliable means for classifying and quantifying early disease in order to evaluate the effectiveness of strategies to prevent disease progression.
Laser microdissection and molecular diagnostics technology development
Integrative molecular biology requires an understanding of interactions of large numbers of pathways. Similarly, molecular medicine increasingly relies on complex macromolecular diagnostics to guide therapeutic choices. A fundamental argument for laser capture microdissection (LCM) of tissues is that, without separation of specific cell populations from complex tissues, we would miss critical control functions of thousands of regulated transcription factors, cell regulators, and receptors that are expressed at low copy number. By detecting changes in these critical effectors, we expect to improve our integrative understanding of tissue function and pathology. In complex tissues—particularly among pathological variations—it is exceptionally difficult to measure the majority of molecules that are at low copy number per cell without first isolating specific cell populations. With our newest inventions, we are increasing the microdissection resolution to the organelle level.
The LCM techniques that we developed are now widely used in molecular analysis of genetics and gene expression changes in target cells within complex tissues. However, in global proteomic and lipid studies without molecular amplification methods, the quantity of isolated cells sufficient to perform accurate characterization of less abundant species is problematic because the microscopic visualization, targeting, and isolation in LCM has a maximal rate of 1–20 cells per second, depending on the cells’ microscopic distribution within the tissues.
Recently, in collaboration with NCI and CIT, we invented and are now refining an automatic "target-directed microtransfer" technique based on macromolecule-specific staining of cells or alternatively organelle-specific staining. The technique (patent pending) is built on our understanding of the physics of thermoplastic microtransfer and uses a much simpler device and transfer films than do commercial laser microdissection microscopes. Given that the new microbonding process does not require microscope-based visualization and targeting, it is capable of much higher throughput rates. Our current prototype is capable of isolating all specifically stained cells within a 1 cm2 region of an immunostained tissue section in about 5 seconds, which corresponds to specific separation from approximately 100,000 cells per second. This technique allows cell separation rates exceeding fluorescence-activated cell sorting (FACS) of labeled cell suspensions while preserving our ability to harvest specifically stained targets directly from sections of complex tissues. This rapid, automated microtransfer method has a spatial resolution determined by stain localization (less than 1 µ), not by optical diffraction. Consequently, it is uniquely suited to isolating highly dispersed, specific cell populations (e.g., stem cells or only those neurons in the supra-optic nucleus that express vasopressin) or specific organelles (e.g., neuronal nuclei in the brain). The spatial relationships (morphology) among the specific cells in the tissue are preserved on the transfer film. Recently, with funding from NIH Director's Challenge Grant, we have joined a multi-institute team to develop and apply expression microdissection to proteomic analysis of specific organelles within tissue sections.
Gene expression during normal development and pathology progression
If microdissection and molecular analysis can be made clinically practical, the expression levels of sets of approximately 20–100 critical, stage-specific disease markers within a selected cell population might provide reliable diagnosis and intermediate endpoints of response to molecular therapies in individual patients. Our analysis of large gene expression and protein databases suggests that a significant fraction of all genes is expressed in any specific cell type and that the levels of gene products universally exhibit a highly skewed power-law distribution similar to those characterizing many other complex systems (Kuznetsov VA, Signal Processsing 2003;83:889). We have developed mathematical models for the evolution of such distributions—models that predict the observed distributions of genes, protein domains, and gene expression in species of increasing biological complexity (Kuznetsov et al., Genetics 2002;161:1321). In collaboration with Jacob Brown and Brian Brooks, we devised a method for using LCM to integrate small regions from the developing eye around the time of topological closure in the mouse embryo for comprehensive gene expression analysis in order to identify candidate genes associated with coloboma, a common human development defect. We are applying dimensionality reduction algorithms to identify temporal classes within Affymetrix® gene expression data from LCM-dissected target tissue for eight distinct time points around the time of normal retina closure. We will then apply such analysis to databases of gene pathway, transcription factor–related expression, and so forth, in order to develop a more integrative understanding of the normal developmental program and extend the list of candidate genes that may be associated with the occurrence of coloboma.
Our research in statistics of less abundant gene products and suitable detection methods using microdissection is guided by a vision of molecular diagnosis evolving from one based on qualitative or quantitative analysis of a few key macromolecules to one in which dimensionality reduction and classification algorithms analyze complex multivariate databases. Such analyses should allow a more complete identification of highly correlated clinical cases and the characterization of their response to molecular therapies specifically designed to prevent progression.
Prevention of progression of age-related macular degeneration through photoprotection
Our clinical studies are designed to test our biophysical model and clinical hypothesis that spectral imbalances of light reaching the retina lead to increased levels of toxic photochemicals within the retinal pigment epithelium, thereby driving early stages of AMD. If our hypothesis is correct, we believe that, during the earliest stages of retinal pathology, this process may be reversible. Therefore, progression to more advanced AMD might be prevented with the use of spectral sunglasses in bright daylight or by otherwise altering the spectrum of ambient light reaching the retina. Using our specifically designed bicolored sunglasses, we have begun non-invasive clinical studies in subjects who have undergone bilateral cataract surgery. Our aim is to image expected changes in the distribution of toxic photochemicals within the human retina. We are improving the autofluorescence imaging protocols and multispectral image analysis in order to provide high-resolution, molecularly specific images of early microscopic changes, thereby allowing us to follow the lesions’ changes over time in relation to photochemicals present in various retinal cell layers. In collaboration with the University of Maryland’s experts in the mathematical analysis of hyperspectral images and clinicians in NEI’s Clinical Branch, we are developing and testing new quantitative tools for following non-invasively the earliest changes in macular pathology in AMD and other retinal diseases. Our goal is to provide better early diagnosis and methods to evaluate early intervention and disease prevention strategies.
In our clinical collaborations at the NIH and with the National Naval Medical Research Center, the Walter Reed Army Medical Center, and the Institute of Eye Diseases in Moscow, we are seeking to apply non-invasive spectral imaging methods to study early photochemical injury and the means of limiting it. Lipofuscin (including undigestible fluorescent photoproducts of the A2E pathway) accumulates with age in the retinal pigment epithelium (RPE) and co-localizes with the acute injury caused by photosensitization of reactive oxygen intermediates (ROI) in the primate retina when exposed to unnaturally high doses of blue light. This observation, along with a variety of epidemiological studies, has led to a widely held hypothesis that cumulative blue-light photochemistry is harmful and plays a role in AMD progression. We have developed an alternative biophysical model of (1) the normal accumulation during aging of potentially damaging photoproducts in the RPE and (2) changes in this “natural” aging associated with external spectral filtering (sunglasses) or cataract surgery with intra-ocular lens implantation. The precursors (principally A2PEH2) of A2E and its oxidation products that segregate within lipofuscin granules in the RPE originate from reactions of all-trans-retinal within the photoreceptor outer segments (ROS) when in bright daylight. Although RPE lysosomal processing enzymatically digests over 99% of shed ROS contents, A2E and related fluorophores are not broken down but rather concentrate into lipofuscin granules. By age 60, the average steady-state concentrations within the RPE reach approximately 200 µM in normal eyes. However, A2E is toxic to cellular membranes at much lower concentrations.
Our novel hypothesis (Meyers et al., Trans Am Ophthalmol Soc 2004;102:83) posits that blue light photosensitizes singlet oxygen generation within lipofuscin granules. The singlet oxygen efficiently oxidizes A2E within the lipofuscin granule in which it was generated and is the principal means of controlling steady-state levels of A2E in the RPE. As short-wavelength macular irradiance decreases with age, the rate of A2E photo-oxidation falls approximately up to 20-fold, causing the steady-state concentration ([A2E]ss) in the normal phakic eye to increase even as rod bleaching and A2E production decrease. Our theoretical model of macular aging reproduces the normal age dependence of lipofuscin and A2E. It provides a primary cytotoxic mechanism in which, once A2E reaches a threshold concentration in the RPE cell, A2E redistribution into critical membranes causes damage with or without additional photoactivation. The model also predicts that, in normal RPE, nearly constant levels of A2E are maintained at a given age and lens color, irrespective of total ambient light exposure. It is primarily the yellowing of the lens with age that distorts the original spectral balance between the rate of production and rate of photo-oxidation found in youth, allowing [A2E]ss to rise with age. If our model is correct, then restoring or optimizing the spectral balance with external spectrally selective sunglasses could significantly lower A2E levels and may prevent associated macular degeneration. Our clinical studies using non-invasive, multispectral autofluorescence imaging to map the levels of fluorescent A2E pathway molecules and other important optical molecules in the retina (e.g., macular pigment, hemoglobin, melanin, unbleached opsins) are designed to develop more effective noninvasive molecular imaging and lesion characterization within the retina with the goal of gaining a better understanding of early disease progression and evaluating the effectiveness of early disease prevention
To this end we have, with NSF support, established a multi-institution effort to develop more effective quantitative image analysis methods capable of characterizing changes in microscopic retinal changes associated with the earliest disease states.
Temporal-spectral programmable LED lighting to optimize health
We are developing test-beds to study health and productivity effects of controlling temporal patterns of blue light (460nm LEDs) that set daily (circadian) physiological rhythms and alertness via nonvisual melanopsin photoreceptors in the retina. Solid-state lighting is a rapidly evolving technology with increasing brightness and energy efficiency and life-cycle cost effectiveness. LEDs concentrate their emissive energy into narrow spectral bands, which can be optimized for human photoreceptor sensitivity. High-power blue LEDs are optimal for activation of recently discovered nonvisual photoreception by melanopsin-containing retinal ganglion cells that project to brain centers that control circadian rhythms and alertness. Lens yellowing with age lowers the amount of blue-light reaching the retina. This puts aging populations spending daylight hours in offices and hospitals with conventional artificial lighting at greater risk for loss of normal diurnal rhythms and alertness. Brain responses to the blue light–sensitive retinal ganglion cells vary greatly with time of day. Optimal wellbeing will require temporal control of artificial light spectrum throughout the day. Although some basic principles of these complex interactions have been revealed by controlled sleep studies, real-living environment test-beds like those we are developing are required to optimize spectral and temporal patterns for improved health and productivity. We have developed models of spectral irradiance as a function of ambient light levels—models that we are modifying for the effects of spectral shifts in our LED light sources, which affect steady-state pupil diameters. As we develop suitable programmable lighting sources in our test-beds, we propose to evaluate the effects of various spectral-temporal lighting patterns on human and animal diurnal rhythms, alertness, mood, and productivity. Supported by a Department of Energy Federal Energy Management Program grant, we shall integrate the expertise of the Lighting Research Division of Lawrence Livermore National Laboratories and their access to commercial partners to insure that improvements in health and wellbeing that are uncovered by our research can be commercially implemented.
United States Patents
- Bonner RF, Goldstein SR, Smith PD, Pohida TJ. Method of laser capture microdissection from a sample utilizing short pulse #6,897,038; May 24, 2005
- Goldstein SR, Bonner RF, Smith PD, Peterson J, Pohida TJ. Mechanical handling systems for laser capture microdissection #6,720,191; April 13, 2004
- Liotta LA, Buck MF, Weiss RA, Zhuang Z, Bonner RF. Isolation of cellular material under microscopic visualization. #6,569,639; May 27, 2003.
- Bonner RF, Goldstein SR, Smith PD, Pohida TJ. Precision laser capture microdissection utilizing short pulse length. #6,420,132; July 16, 2002.
- Bonner RF, Liotta L, Buck M, Krizman DB, Chuaqui R, Linehan WM, Trent JM, Goldstein SR, Smith PD, Peterson JI. Isolation of cellular material under microscopic visualization. #6,251,516; June 26, 2001.
- Bonner RF, Pohida T, Buck M, Tangrea M, Chuaqui R. Target-directed microtransfer; patent pending.
Additional Funding
- NSF Biophotonics 0854233 Multispectral Retinal Imaging and Mapping of Naturally Occurring Fluorophore and Chromophore Distributions in Health and Early Pathology
- DOE FEMP Testbeds with temporal-spectral programmable LED lighting to optimize health, well-being and productivity in studies of workers and patients at the National Institutes of Health
Publications
- Brown JD, Dutta S, Bharti K, Bonner RF, Munson PJ, Dawid IB, Akhtar AL, Onojafe IF, Alur RP, Gross JM, Hejtmancik JF, Jiao X, Chan WY, Brooks BP. Expression profiling during ocular development identifies 2 Nlz genes with a critical role in optic fissure closure. Proc Natl Acad Sci USA 2009 106:1462-1467.
- Chan CC, Ross RJ, Shen DF, Ding XY, Majumdar Z, Bojanowski CM, Zhou M, Salem N, Bonner R, Tuo JS. Ccl2/Cx3cr1-deficient mice: an animal model for age-related macular degeneration. Ophthalmic Res 2008 40:124-128.
- Wong WT, Forooghian F, Majumdar Z, Bonner RF, Cunningham D, Chew EY. Fundus autofluorescence in type 2 idiopathic macular telangiectasia: correlation with optical coherence tomography and microperimetry. Am J Ophthalmol 2009 148:573-583.
Collaborators
- Sergei Avetisov, MD, Institute of Eye Diseases, Moscow, Russia
- David Berler, MD, Washington Eye Physicians & Surgeons, Chevy Chase, MD
- Brian Brooks, MD, PhD, Ophthalmic Genetics and Visual Function Branch, NEI, Bethesda, MD
- Jacob Brown, MD, PhD, Ophthalmic Genetics and Visual Function Branch, NEI, Bethesda, MD
- Emily Chew, MD, Clinical Branch, NEI, Bethesda, MD
- Denise Cuningham, CRA, RBP, MEd, Clinical Branch, NEI, Bethesda, MD
- Wojciech Czaja, PhD, Norbert Weiner Center, University of Maryland, College Park, MD
- Francisco De Monasterio, MD, PhD, Clinical Branch, NEI, Bethesda, MD
- Tatiana Kiseleva, MD, DSc, Institute of Eye Diseases, Moscow, Russia
- Jennifer Lippincott-Schwartz, PhD, Cell Biology and Metabolism Program, NICHD, Bethesda, MD
- Sanford P. Markey, PhD, Laboratory of Neurotoxicology, NIMH, Bethesda, MD
- David O. Mazur, MD, Integrated Ophthalmology Service, National Naval Medical Center, Bethesda, MD
- Philip G. McQueen, PhD, Mathematical and Statistical Computing Laboratory, CIT, Bethesda, MD
- Sanford Meyers, MD, Retina Consultants, Des Plaines, IL
- Tom Pohida, MSE, Computational Biology and Electronics Laboratory, CIT, Bethesda, MD
- Wai T. Wong, MD, PhD, Ophthalmic Genetics and Visual Function Branch, NEI, Bethesda, MD
- Barry R. Zeeberg, PhD, Laboratory of Molecular Pharmacology, NCI, Bethesda, MD
Contact
For more information, email bonnerr@mail.nih.gov.