You are here: Home > Section on Medical Biophysics
Medical Biophysics
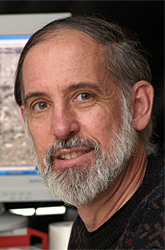
- Robert F. Bonner, PhD, Head, Section on Medical Biophysics
- Martin Ehler, PhD, Visiting Fellow
- Emily J. King, PhD, Postdoctoral Fellow
- Zigurts Majumdar, PhD, Special Volunteer
- Sanford Meyers, MD, Guest Researcher
- Kimberly Tran, MS, Technical Training Fellow
We develop new optical technologies in order to characterize or modify early stressors that drive chronic diseases and use them in developing effective disease-prevention strategies. Through integrated analysis of multispectral, multimodal clinical retinal imaging, we map distributions and dynamics of retinal photochemicals and relate them to cellular dysfunction and early disease progression. By applying these new methods in clinical studies, we seek to test our hypothesis that spectral shifts in retinal irradiance can reduce imbalances among retinal photochemical pathways. We posit that chronic photochemical imbalances drive early age-related and Stargardt's maculopathies, which could be reduced or prevented by appropriate external filters (e.g., spectral sunglasses). Our noninvasive molecular mapping methods might improve characterization of early retinal disease states including more readily reversible "preclinical" disease and could also be used to measure the effects of benign, low-cost preventions strategies. We also are adapting our prior inventions of laser capture microdissection (LCM) into simpler systems more easily integrated with clinical pathology and multiplex molecular analysis of targeted cells and organelles extracted from complex tissue.
Laser microdissection and molecular diagnostics technology development
Integrative molecular biology requires an understanding of interactions of large numbers of pathways. Similarly, molecular medicine increasingly relies on complex macromolecular diagnostics to guide therapeutic choices. A fundamental argument for LCM of tissues is that, without separating specific cell populations from complex tissues, we would miss critical control functions of thousands of regulated transcription factors, cell regulators, and receptors that are expressed at low copy number. By detecting changes in these critical effectors, we expect to improve our integrative understanding of tissue function and pathology. In complex tissues—particularly among pathological variations—it is exceptionally difficult to measure the majority of molecules that are found at low copy number per cell without first isolating specific cell populations. The LCM techniques that we developed are now widely used in molecular analysis of genetics and gene expression changes in target cells within complex tissues.
In global proteomic, lipid, and other studies without robust molecular amplification methods, the quantity of isolated cells sufficient to perform accurate characterization of less abundant species in typical LCM samples is problematic. The low throughput, high cost, and complexity of microscopic visualization, targeting, and isolation in LCM has also limited its incorporation into routine clinical diagnostics. To address these limitations, in collaboration with NCI and CIT, we invented (US Patents #7,709,047 and #7,695,752) target-activated microdissection (TAM), in which absorptive histochemical stains determine sites of microbonding to a flexible overlying thermoplastic film. When using stains specific for biomarker molecules (e.g., immunohistochemistry), the TAM variant called expression microdissection (xMD) permits rapid, automatic microtransfer of specifically labeled cells or organelles (nuclei) from formalin-fixed paraffin-embedded (FFPE) animal and clinical tissue sections. In parallel with developing proteomic applications using our laser-scanning prototype, we developed this year a very simple flashlamp system for instantaneous microbonding to all specific targets within a large sample area. The new, low-cost instrument design builds on our understanding of the physics and dosimetry to achieve ultra-precise capture using 1 micron–thick thermoplastic polymer coating on a flexible PET tape. Using a flashlamp-based activation of the flexible transfer films held in physical contact with the tissue surface in a specially designed slide holder, we reliably captured prostate cancer nuclei and neuronal nuclei from fixed paraffin-embedded tissue sections with separation at the nuclear envelope. Scanning electron microscopy of such nuclear transfers supports our computer simulations of the process of local polymer melting and precise bonding with targeted nuclei. The combination of submillisecond flashlamp pulses and a 1-micron thermoplastic layer in contact with the specifically stained targets insures high lateral precision over a significant range of light doses. This new simple, low-cost, high-throughput TAM appears well suited for multiplex molecular analyses in clinical pathology. With funding from an NIH Director's Challenge Grant, a multi-institute team (NICHD, NIMH, NIBIB, NCI, and CIT) adapted our xMD methods for mass-spectrometric proteomic analysis of specific neural nuclei within FFPE sections of rat brain.
Gene expression during normal development and pathology progression
Our previous analysis of large gene-expression and protein databases suggests that a significant fraction of all genes are expressed in each cell phenotype and that the copy number per cell of all gene products universally exhibits a highly skewed power-law distribution. Consequently most gene products exist at very low copy-number per cell, and their pathway associations and critical roles may not be detectable in tissue or cytology samples with mixed cell phenotypes or microenvironments without the use of microdissection. As we refine our microdissection technologies towards small-footprint, easy-to-use devices suitable for commercialization, we have sought to develop new insights into the potential for integrating target-specific microtransfers with highly multiplex macromolecular analyses. Embryonic development relies on precise patterns of spatial temporal patterns of gene regulation. In collaboration with the NEI, we adapted LCM to isolate localized (3D) cells at the site of retinal topological closure and performed microarray analysis of gene expression at eight time points of embryonic development, permitting identification of low-copy-number transcriptions factors that, when blocked, lead to loss of closure in animal models. The transcription factors and approximately 200 other temporally and spatially covariant genes appear likely to play a role in coloboma, a inborn developmental defect of the human retina. To improve analysis of this large expression dataset, we developed some new mathematical approaches to identify, within microdissected tissues, networks of genes that drive spatio-temporal changes. We used nonlinear gene clustering with labeling of selected known associations to classify associations among 3,000 genes showing significant temporal variations. Using the functionality tool GoMiner, we evaluated novel clustering algorithms that appear to better characterize interacting pathways of expression associated with specific spatio-temporal programs during development. The methods appear to be particularly useful for identifying new associations among and within functional pathways and could assist in uncovering the integrative biology of spatio-temporal patterns.
Prevention of progression of age-related macular degeneration through photoprotection
Age-related macular degeneration (AMD) is the most common cause of blindness in the aged U.S. population. Stargardt's dystrophy is an genetically-linked retinal disease that causes severe visual loss at much younger ages in a manner akin to rapid geographic atrophy in AMD. Local autofluorescence changes in AMD and Stargardt's dystrophy suggest that increased levels of retinal fluorescent bisretinoids within retinal pigment epithelial (RPE) cause RPE dysfunction and drive early disease progression. Our biophysical model predicts that the steady-state levels of A2E, the bisretinoid with greatest apparent cytotoxicity, are principally determined by the balance between green light that activates rhodopsin and shorter wavelength blue-violet light that photochemically drives bisretinoid detoxification via singlet oxygen reactions localized within the RPE lipofuscin. According to our hypothesis, changes in spectral transmission of the lens with age (i.e., lens yellowing) create a progressive photochemical pathway imbalance that causes A2E levels to rise to levels and that ultimately leads to progressive RPE dysfunction. We believe that spectral photoprotection of retinal rod rhodopsin when in bright daylight (e.g., with vermillion sunglasses) should significantly reduce bisretinoid production and the accumulated RPE and photoreceptor stress. We designed spectral sunglasses that reduce rhodopsin activation 30-fold without significant reduction in photopic sensitivity or color perception. Recently, visual cycle inhibitors that slow rhodopsin turnover up to 10-fold in both day and night have begun clinical trials. However, such inhibitors have obvious side effects (e.g., slowed dark adaptation and reduced rod sensitivity). These and other adverse effects of long-term reductions in the visual cycle are avoided with spectral sunglasses, which are used only in daylight when rhodopsin turnover rates are high and thus likely to create bisretinoids.
An alternative photochemical hypothesis is that violet light photochemistries within the retina in the presence of higher levels of retinaldehydes and accumulated bisretinoids induce chronic photo-oxidative stress from longer-lived reactive oxygen species. Such violet-blue light–driven photochemistries can be greatly reduced by yellow sunglass filters. To test these alternative hypotheses, we designed bicolored sunglasses in which one eye is provided with a vermilion (green-blocking) filter that specifically protects rhodopsin and the other eye with a yellow (blue-violet–blocking) filter that specifically protects against short-wavelength photochemical injury. Such sunglasses allow us to compare short-term photochemical changes between eyes in which only spectral irradiance is changed while genetics, physiology, and environmental exposures otherwise remain constant.
In our clinical studies, we are seeking to test predictions of our biophysical model and disease hypothesis, i.e., that spectral imbalances in retinal illuminance raise levels of toxic photochemicals in the RPE, which lead to progressive dysfunction in early stages of AMD and other retinal diseases associated with RPE dysfunction. All bisretinoids are fluorescent molecules with distinct excitation and emission spectra. By using fundus cameras adapted for multispectral autofluorescence imaging, we are attempting to detect and follow predicted changes in the bisretinoid pathway between the paired eyes. If correct, we believe that, at the earliest stages of retinal pathology, this imbalance may be reversed and progression to more advanced AMD and that visual loss might be reduced by wearing external spectral sunglasses or otherwise altering the spectrum of the ambient light that reaches the retina. Our clinical autofluorescence multispectral imaging would then be used to compare photochemical changes within local retinal lesions having observable RPE dysfunction (e.g., drusen) with nearby more normal-appearing retina. Such image analysis could lead to more accurate understanding of early lesion progression and to a method to measure restoration of function.
Recently, we developed a new noninvasive imaging method to map, with about 30 micron resolution, rhodopsin densities in patients. Confocal scanning laser ophthalmoscope (cSLO) at 488nm or 515nm permits continuous autofluorescence imaging at low-light doses that only bleach about 1% of the rod rhodopsin per image. After 25–40 sec of imaging, the rhodopsin bleaching achieves steady state in which more than 95% of the rhodospin is "bleached" (activated rhodopsin form in which absorption peak at 507nm is lost). The normal optical density of the photoreceptor layer is about 0.3 at 488–515nm in the initial partially dark-adapted subject and consequently reduces the laser light reaching the fluorescent bisretinoids in the RPE by about 50%. By registering and determining the ratio of the cSLO images sequence of dark-adapted to bleached rhodopsin states, we can obtain a high-resolution map of rhodopsin density (pixel size of about 10 micron). In preliminary results at the NEI Clinic, we observed local loss of rhodopsin within both hypo- and hyperfluorescent lesions in a Stargardt's patient. This apparent loss of bleachable rhodopsin was seen in regions with both abnormal and normal photoreceptor layer indicated by optical coherence tomography (OCT). Thus, the new imaging modality may offer unique high-resolution functional imaging of the rod rhodopsin and its local regeneration by the visual cycle. In collaboration with the NEI Clinic, we are seeking to correlate local variations in retinal autofluorescence with local changes in rhodopsin density within regions of apparent AMD and Stargardt's pathology. Such noninvasive studies may help clarify the differences between reticular drusen and subRPE drusen and their respective rates of progressive retinal damage.
In collaboration with applied mathematicians at the University of Maryland, we are seeking to develop new quantitative analysis tools for multimodal retinal image sets that could identify and monitor earlier changes in macular pathology in AMD and a wider variety of retinal diseases. Such methods could provide better statistical characterization of early disease states and thus might permit improved studies of early intervention for disease prevention.
Temporal-spectral programmable LED lighting to optimize health
Modern life is associated with 24-7 activities made possible by ubiquitous use of artificial lighting. Demonstration of nonvisual retinal photoreception via blue-light (460-480nm peak) activation of melanopsin within a subset of retinal ganglion cells led to the recognition that average blue-light irradiance levels play a critical role in attention and synchronization of circadian physiology. Human evolution has developed such optimized controls based on normal variations in retinal irradiance associated with the diurnal solar cycle. Modern life and ubiquitous artificial light sources have greatly modified temporal patterns in retinal irradiance, which may have such diverse health effects as increased incidence of cancer, metabolic syndrome, sleep disturbances, and possibly a wide spectrum of neuro-psychiatric disorders, particularly attention deficit and depressive disorders. Conversely natural seasonal variations in daylight periods can induce seasonal affective disorder, which responds to early morning artificial blue-light enrichment. Advances in more energy-efficient and spectrally specific light sources such as light-emitting diodes (LEDs) offer new opportunities for optimization of the spectral composition of artificial lighting that could have a significant impact on biological function and health. We are interested in how we can study the effects of modern lighting on health and performance in ways that might inform new lighting standards to guide commercial lighting developments.
Sunlight acting through the mc-RGC (melanopsin-containing retinal ganglion cells) pathway profoundly affects both acute brain function and daily entrainment of circadian physiology. Modern architectural design emphasizes the use of natural sunlight where possible. Night-time exposures to artificial lighting can be disruptive of circadian physiology, as shown by numerous studies on shift-workers, particularly irregular shift work. As a consequence, one might improve health and productivity of the modern populace in general by optimizing spectral-temporal control of artificial lighting that at night balances photopic visual acuity for night-time tasks with minimizing circadian disruption by blue-light. Conversely, enriching daytime blue light components in artificial lighting may improve acute brain functions such as alertness and prevent circadian disruptions in individuals who are indoors during most daylight hours. Activation of melanopsin in the mc-RGCs by artificial lighting that is sufficient during the daytime and minimal at night requires a paradigm shift for the lighting industry.
Our current knowledge of human non-visual effects of spectral lighting is largely limited to isolated controlled sleep-lab studies on small numbers of subjects and risk factors in large-scale epidemiology studies. Even with significant new data about the action spectra of the mc-RGC cells (including blue-red photoreversal) and their role in important brain pathways, including acute alertness and circadian synchronization, we do not have a rational approach to designing optimal spectral lighting for health and productivity because of the difficulties in testing real-world effects of spectral temporal lighting for human performance and physiology. Common artificial light sources that contribute the highest retinal irradiance are computer monitors (also smart phones and televisions) universally designed to exceed ambient light levels reaching the retina. In our modern society, large segments of the population average four hours of computer use, and the typical household television is on for eight hours a day. These high-brightness sources on which we routinely fixate for long periods are therefore most likely to alter natural patterns of activation of melanopsin-containing retinal ganglion cells and their projections to the brain. We believe that there are significant numbers of individuals whose spectral-temporal retinal irradiance from their daily computer use (and other personal electronic devices with luminous screens) puts them at risk for circadian disruption and a variety of associated physiological stresses ( contributing, e.g., to the rise in metabolic syndrome). With assistance from the DOE and the Lighting Division of Lawrence Berkeley National Laboratory, we have developed software that controls RGB (red, green, blue) color balance of LED/LCD screen displays independently of the computer's normal operational programs. Based on published melanopsin spectral sensitivity, we can currently dynamically modulate melanopsin activation over a critical 10-fold range while maintaining photopic sensitivity and color-rendering. We are adapting this spectral-temporal testbed for effects on acute cognitive function and alertness in a format that could be readily exported for typical office and home computer use. If we can devise reliable measures of spectral-temporal optimization, we envision developing freeware that could enable individuals to self-test and determine individual acute optimization with eventual extension to diurnal data logging for individual optimization of circadian physiology.
United States Patents
- Emmert-Buck MR, Tangrea MA, Bonner RF, Chuaqui R, Pohida TJ. Target activated microtransfer. #7,709,047; May 4, 2010.
- Bonner RF, Pohida TJ , Emmert-Buck MR, Tangrea MA, Chuaqui R,. Target activated microtransfer. #7,695,752; April 13, 2010.
- Bonner RF, Goldstein SR, Smith PD, Pohida TJ. Method of laser capture microdissection from a sample utilizing short pulse. #6,897,038; May 24, 2005.
- Liotta LA, Emmert-Buck M, Krizman DB, Chuaqui R, Linehan WM, Trent JM, Bonner RF, Goldstein SR, Smith PD, Peterson JI. Isolation of cellular material under microscopic visualization. #6,867,038; March 15, 2005.
- Bonner RF, Goldstein SR, Smith PD, Pohida TJ. Non-contact laser capture microdissection. #6,743,601; June 1, 2004.
- Goldstein SR, Bonner RF, Smith PD, Peterson J, Pohida TJ. Mechanical handling systems for laser capture microdissection. #6,720,191; April 13, 2004
- Liotta LA, Buck MF, Weiss RA, Zhuang Z, Bonner RF. Isolation of cellular material under microscopic visualization. #6,569,639; May 27, 2003.
- Bonner RF, Goldstein SR, Smith PD, Pohida TJ. Precision laser capture microdissection utilizing short pulse length. #6,420,132; July 16, 2002.
- Bonner RF, Liotta L, Buck M, Krizman DB, Chuaqui R, Linehan WM, Trent JM, Goldstein SR, Smith PD, Peterson JI. Isolation of cellular material under microscopic visualization. #6,251,516; June 26, 2001.
Additional Funding
- NSF Biophotonics 0854233 (2009-2011) Multispectral Retinal Imaging and Mapping of Naturally Occurring Fluorophore and Chromophore Distributions in Health and Early Pathology
- DOE FEMP (2010) Temporal-spectral programmable LED lighting: Test-beds for health and sustainability.
- NIH Director's Challenge Award (2009-2010) Subcellular microdissection for the identification of organelle proteins
Publications
- Ehler M, Rajapakse V, Zeeberg B, Brooks B, Brown J, Czaja W, Bonner RF. Analysis of Temporal-spatial Covariation within Gene Expression Microarray Data in an Organogenesis Model. Bioinformatics Research and Applications. LNICS 2010;6051:38-49.
- Ehler M, Rajapakse VN, Zeeberg BR, Brooks BP, Brown J, Czaja W, Bonner RF. Nonlinear gene cluster analysis with labeling for microarray gene expression data in organ development. BMC Proc 2011;5S2:S3.
- Hanson JC, Tangrea MA, Kim S, Armani MD, Pohida TJ, Bonner RF, Rodriguez-Canales J, Emmert-Buck MR. Expression microdissection adapted to commercial laser dissection instruments. Nat Protoc 2011;6:457-467.
- Tangrea MA, Hanson JC, Bonner RF, Pohida TJ, Rodriguez-Canales J, Emmert-Buck MR. Immunoguided microdissection techniques. Methods Mol Biol 2011;755:57-66.
- Zeeberg BR, Liu H, Kahn AB, Ehler M, Rajapakse VN, Bonner RF, Brown JD, Brooks BP, Larionov VL, Reinhold W, Weinstein JN, Pommier YG. RedundancyMiner: De-replication of redundant GO categories in microarray and proteomics analysis. BMC Bioinformatics 2011;12:52.
Collaborators
- Brian Brooks, MD, PhD, Ophthalmic Genetics and Visual Function Branch, NEI, Bethesda, MD
- Emily Chew, MD, Clinical Branch, NEI, Bethesda, MD
- Denise Cuningham, CRA, RBP, MEd, Clinical Branch, NEI, Bethesda, MD
- Wojciech Czaja, PhD, Norbert Weiner Center, University of Maryland, College Park, MD
- Michael M. Emmert-Buck, MD, PhD, Laboratory of Pathology, NCI
- William K. Floyd, Division of Environmental Protection, OD, NIH, Bethesda, MD
- Jennifer Lippincott-Schwartz, PhD, Cell Biology and Metabolism Program, NICHD, Bethesda, MD
- Sanford P. Markey, PhD, Laboratory of Neurotoxicology, NIMH, Bethesda, MD
- Philip G. McQueen, PhD, Mathematical and Statistical Computing Laboratory, CIT, Bethesda, MD
- Sanford Meyers, MD, Retina Consultants, Des Plaines, IL
- Nicole Y. Morgan, PhD, Laboratory of Bioengineering and Physical Science, NIBIB, Bethesda, MD
- Tom Pohida, MSE, Computational Biology and Electronics Laboratory, CIT, Bethesda, MD
- Francis M. Rubinstein, PhD, Lawrence Berkeley National Laboratory, Berkeley, CA
- Wai T. Wong, MD, PhD, Ophthalmic Genetics and Visual Function Branch, NEI, Bethesda, MD
- Barry R. Zeeberg, PhD, Laboratory of Molecular Pharmacology, NCI, Bethesda, MD
Contact
For more information, email bonnerr@mail.nih.gov.