You are here: Home > Section on Molecular Endocrinology
Receptors and Actions of Peptide Hormones and Regulatory Proteins in Endocrine Mechanisms
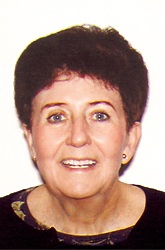
- Maria L. Dufau, MD, PhD, Head, Section on Molecular Endocrinology
- Chon-Hwa Tsai-Morris, PhD, Staff Scientist
- Mingjuan Liao, PhD, Staff Fellow
- Junghoon Kang, PhD, Postdoctoral Fellow
- Raghuveer Kavarthapu, PhD, Postdoctoral Fellow
- Joaquin Villar, PhD, Postdoctoral Fellow
- Lisheng Dai, Predoctoral Fellow
We investigate the molecular basis of peptide hormone control of gonadal function, with particular emphasis on the structure and regulation of the genes encoding the luteinizing hormone receptor (LHR) and prolactin (PRL) receptor (PRLR). We also investigate the regulatory mechanism(s) involved in the progress of spermatogenesis and the control of Leydig cell (LC) function. Our studies focus on the regulation of human LHR transcription (nuclear orphan receptors, epigenetics, DNA methylation, and second messengers, repressors, corepressors, and coactivators), as well as on the multiple-promoter control of hPRLR gene transcription. We are elucidating the functions of two inhibitory short forms of prolactin receptors and their impact on the long form of the receptor as well as their relevance to physiological regulation and breast cancer. We also investigate novel gonadotropin-regulated genes of relevance to the progression of testicular gametogenesis, Leydig cell function, and other endocrine processes. We concentrate our studies on the function and regulation of gonadotropin-regulated testicular RNA helicase (GRTH/DDX25), an essential post-transcriptional regulator of spermatogenesis that was discovered, cloned, and characterized in our laboratory. The various functions of GRTH/DDX25 provide a fertile ground for the development of a male contraceptive.
The luteinizing hormone receptor
The luteinizing hormone receptor (LHR) is a G-coupled seven-transmembrane receptor, which is expressed primarily in the gonads, where it mediates luteinizing hormone signals that regulate cyclic ovarian changes or testicular function. Our earlier findings demonstrated that LHR gene expression at the transcriptional level is regulated by complex and diverse networks, in which coordination and interactions between the regulatory effectors are essential for silencing/activation of LHR expression. The proximal Sp1 site of the LHR promoter recruits histone deacetylases and the Sin3A corepressor complex that contribute to the silencing of LHR transcriptional expression. Site-specific acetylation/methylation induce cell-specific phosphatase release, which serves as an "on" mechanism for Sp1 phosphorylation by PI3K at Ser641, causing p107 repressor de-recruitment from Sp1 and LHR transcriptional activation. Maximal derepression of the LHR gene is dependent on complete DNA demethylation of the promoter, histone hyperacetylation, and the release of repressors (p107 and HDAC/Sin3A), causing recruitment of TFIIB and Pol II and transcriptional activation. Positive Cofactor 4 (PC4) has an important role in the formation/assembly of the preinitiation complex (PIC) in LHR transcription (1). It is recruited by Sp1 following trichostatin A (TSA) treatment and acts as its coactivator. However, PC4 does not participate in TSA release of phosphatases, Sp1 phosphorylation, or release of repressor/complexes. Although TFIIB recruitment is dependent on PC4, we have ruled out TFIIB as its direct target and acetylation of PC4 in the activation process. However, TSA–induced acetylation of a PC4–interacting protein (16 kDa), identified as acetylated H3 by mass spectrometry, and its presence in the complex in association with chromatin at the promoter was demonstrated by ChiP/reChiP. The role of these interactions on chromatin structure and their participation in the assembly of the PIC and transcriptional activation are under investigation.
Gonadotropin-regulated testicular RNA helicase
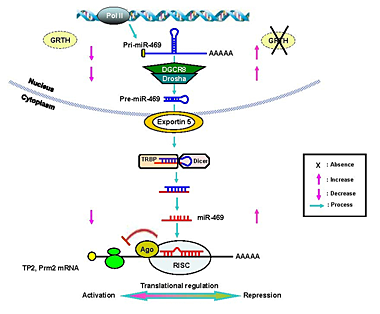
Click image to enlarge.
Figure 1. Model of a proposed mechanism of GRTH regulation on miR-469 biogenesis that represses Transition Protein 2 (TP2) and Protamine 2 (Prm2) gene translation during spermatogenesis
GRTH plays a critical role in maintaining homeostasis of a subset of mature miRNAs by regulating miRNA biogenesis via Drosha-DGCR8 microprocessor complex activation. Absence of GRTH in KO mice up-regulates pri-miR-469 and the Drosha-DGCR8-complex. pre-miR-469 transfers to cytoplasmic sites, where it is further processed via Dicer-dependent pathways to generate mature miR-469. miRNA-469 silences TP2 and Prm2 by its association at the coding region through RNA-induced silencing complex. Such regulation ultimately controls the temporal progression of spermatogenesis via miRNA-469's inhibitory action on TP2/Prm2 protein expression, which is essential for the proteins' timely expression, leading to chromatin compactation in spermatids and the progress of spermatogenesis (3).
Gonadotropin-regulated testicular RNA helicase (GRTH/DDX25) is a testis-specific member of the DEAD–box family of RNA helicases present in LCs and meiotic germ cells. It is a multi-functional protein that is essential for the completion of spermatogenesis. Males lacking GRTH are sterile because round spermatids fail to elongate. Normal basal levels of testosterone in serum and LCs exclude abnormal steroidogenesis as responsible for the arrest of spermiogenesis. In addition to its intrinsic RNA helicase activity, GRTH is a shuttling protein that exports specific mRNAs relevant to spermatogenesis from the nucleous to cytoplasmic sites of germ cells including to polyribosomes and the chromatoid body (CB) of spermatids.
Our recent studies revealed the essential participation of the GRTH export/transport of mRNAs in the structural integrity of the CB (storage/processing of mRNAs) and their transit/association to actively translating polyribosomes, where the helicase may regulate translational initiation of genes. We identified mRNAs that are associated with GRTH and regulated at polysomal sites of cell populations of the mouse testis. In studies comparing GRTH knockout (KO) with wild-type (WT), the reduction in mRNAs we observed associated with abolition of protein expression at these sites—detected in the cytoplasm but not at the total cellular level—reflects the importance of the transport function of GRTH to relevant sites and underscores its impact in protein synthesis. We found messages regulated in different testicular cells associated with GRTH at polysomes. From these studies we derived databases for GRTH–regulated genes in different testicular cells associated with GRTH at polysomes (Geo #GSE38860). Network analysis provided information about regulatory pathways that link to GRTH function (ubiquitin-proteosome-heat shock protein network pathways and NFĸB/TP53/TGFB1 signalling networks). Our studies thus revealed known and unexplored factors and provided fertile ground for exploration of GRTH regulation in the progress of spermatogenesis (2).
In other work, we explored GRTH regulation of miRNAs in round spermatids. Differential expression profiles from WT and GRTH KO mice revealed a panel of miRNAs and their primary miRNAs that were significantly elevated in KO. A database for GRTH–regulated miRNA derived from these studies can be accessed at Geo #GSE33969. Testis-specific upregulated miRNA-469 repressed the translation of Transition Protein 2 (TP2) and Protamine 2 (Prm2) through binding to their mRNA coding region, consistent with the preservation of TP2 and Prm2 mRNA expression and failure of their protein expression in KO. Thus, GRTH has an important role in microRNA regulation, acting as a negative regulator of miRNA-469 biogenesis and expression levels of DROSHA/DGCR8 (mRNA/protein). miRNA-469 silencing of TP2 and Prm2 (chromatin remodelers) is essential for their timely translation at later stages of spermiogenesis, which is critical to attain mature sperm (Figure 1) (3).
GRTH is regulated by LH through androgen (A) at the transcriptional level in LCs (direct) and germ cells (presumably indirect) of the testis, where its expression is both cell- and stage-specific. The helicase displays a novel negative autocrine control of A production in LCs by preventing overstimulation of the LH–induced A pathway through enhanced degradation of the StAR protein. Our initial studies using transgenic mice carrying sequential deletions of 5′ flanking sequences defined an A-responsive l.4 kb region adjacent to an ATG codon that contains an androgen response element (ARE) half-site at −827. Initially, we studied the transcriptional regulation/expression of GRTH by A using an in vitro model (GT1–7 hypothalamic cell line expressing GRTH stably expressing the androgen receptor [AR] at levels comparable to mouse LC) and confirmed in vivo (mouse LC in primary cultures; and LC from mice treated +/− hCG, +/− AR antagonist). In both cases, the GRTH expression induced by A or hCG (via A) was inhibited by the AR antagonist. Deletion mapping and mutagenesis demonstrated that the ARE was critical for dihydrotestosterone (DHT)-induced activation and that it recruited AR in a DHT-dependent manner. ChiP analysis revealed recruitment of AR, steroid coactivator 1 (SRC-1), mediator 1 (Med-1), TFIIB, and Pol II to both the ARE and a region around the initiator sites at the promoter. ChiP-3C revealed short-range chromosomal looping between the distal AR/ARE and the core transcriptional machinery at the promoter. Med-1 and SRC-1-were not required for looping but were essential for the activation of the complex. These findings provided new insights into the molecular mechanism of AR–regulated transcription of the GRTH gene in LCs (Figure 2) (4).
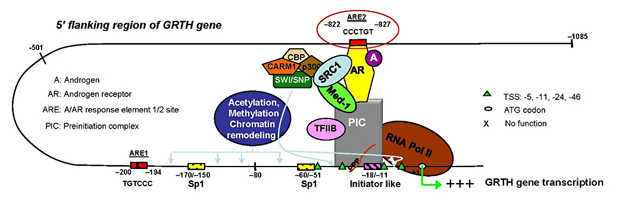
Click image to enlarge.
Figure 2. Model of androgen (A) action on GRTH gene transcription in mouse Leydig cells
In vivo and in vitro, A activates the androgen receptor (AR) (A/AR). The A/AR binds to the functional ARE2 half-site at −827/−822 and interacts with a member(s) of the PIC and Pol II through short-range chromosomal looping. SRC-1 and Med 1, which associate with the AR, are not required for loop formation but are essential for functional activation of transcription of the loop complex of GRTH gene induced by A (4).
The prolactin receptor
The prolactin receptor (PRLR) is a member of the lactogen/cytokine receptor family and mediates the diverse cellular actions of prolactin (PRL). PRL is a major factor in the proliferation and differentiation of breast epithelium and is essential for stimulation and maintenance of lactation. It has been also implicated in the development of breast cancer, tumor growth, and chemoresistance. In the human, hPRLR expression is controlled at the transcriptional level by multiple promoters (one generic, [PIII], and five human specific [hPN1-hPN5]), which were defined and characterized in our laboratory. Each promoter directs transcription/expression of a specific non-coding exon 1 (E1-3, hEN1-hEN5), a common non-coding exon 2, and coding exons (E3-E11). The transcription of PRLR in breast cancer cells by the preferentially utilized PIII, which lacks an estrogen-responsive element, is directed by estradiol (E2)/ERa (estrogen receptor a) through complex formation with SP1 and C/EBPb that associate with cognate elements, inducing TFIIB and Pol II recruitment. Combining bioluminescence resonance energy transfer (BRET) with a bioluminescence resonance complementation assay, we revealed that ERa exists as constitutive homodimers and that E2 induced a change(s) in an ERa homodimer conformation favorable for interaction with the transcription factors Sp1 and C/EBPb. E2-enhanced complex formation of the ERa dimer with SP1 and C/EBPb dimers at the PIII promoter plays an essential role in the transcriptional activation of the hPRLR gene in breast cancer cells (5).
In further studies, we investigated the effect of E2 and testosterone on the expression of various exon 1 isoforms (hE1-3, hEN1-hEN5) transcribed under the control their specific promoters in MCF-7 cells. Real-time PCR of exons 1 showed that E2 stimulated the expression of all exon 1 isoforms except hE1N2, an effect that was prevented by ER antagonist. Exogenous testosterone showed comparable stimulation, which was prevented by an aromatase inhibitor, confirming the endogenous estrogen action in PRLR expression. Changes on PRLR protein expression and cell proliferation displayed similar stimulatory patterns. Current studies are addressing homologous effects of PRL on PRLR through individual promoters. Investigations on PRLR promoter usage and their regulation under hormonal treatment may provide insights into resistance states of breast cancer patients under adjuvant therapies.
Publications
- Liao M, Zhang Y, Kang JH, Dufau ML. Coactivator function of positive cofactor 4 (PC4) in Sp1-directed luteinizing hormone receptor (LHR) gene transcription. J Biol Chem 2011;286:7681-7691.
- Tsai-Morris CH, Sato H, Gutti R, Dufau ML. Role of gonadotropin-regulated testicular RNA helicase (GRTH/Ddx25) on polysomal associated mRNAs in mouse testis. PLoS One 2012;e32470:1-16.
- Dai L, Tsai-Morris CH, Sato H, Villar J, Kang JH, Dufau ML. Testis-specific miRNA-469 up-regulated in gonadotropin-regulated testicular RNA helicase (GRTH/DDX25) transition protein 2 and protamine messages at sites within coding region: implications of its role in germ cell development. J Biol Chem 2011;286:44306-44318.
- Villar J, Tsai-Morris CH, Dai L, Dufau ML. Androgen-induced activation of gonadotropin-regulated testicular RNA helicase (GRTH/Ddx25) transcription: essential role of a nonclassical androgen response element half-site. Mol Cell Biol 2012;32:1566-1580.
- Kang JH, Tsai-Morris CH, Dufau ML. Complex formation and interactions between transcription factors essential for human prolactin receptor gene transcription. Mol Cell Biol 2011;31:3208-3222.
Contact
For more information, email dufau@helix.nih.gov.