You are here: Home > Section on Sensory Coding and Neural Ensembles
Olfactory Coding and Decoding by Ensembles of Neurons
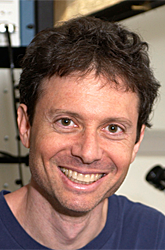
- Mark Stopfer, PhD, Head, Section on Sensory Coding and Neural Ensembles
- Zane Aldworth, PhD, Postdoctoral Fellow
- Nitin Gupta, PhD, Postdoctoral Fellow
- Takaaki Miyazaki, PhD, Postdoctoral Fellow
- Kazumichi Shimizu, PhD, Postdoctoral Fellow
- Samuel Reiter, MS, Graduate Student
- Kui Sun, MD, Technician
All animals need to know what is going on in the world around them. Thus, brain mechanisms have evolved to gather and organize sensory information in order to build transient and sometimes enduring internal representations of the environment. Using relatively simple animals and focusing primarily on olfaction and gustation, we combine electrophysiological, anatomical, behavioral, computational, genetic, and other techniques to examine the ways in which intact neural circuits, driven by sensory stimuli, process information. Our work reveals basic mechanisms by which sensory information is transformed, stabilized, and compared, as it makes its way through the nervous system.
A temporal channel for information in sparse sensory coding
Brain circuits encode sensory information into a variety of neural representations ranging from dense, time-varying patterns of spikes in overlapping sets of neurons to sparse spikes in a few selective neurons. Sparse codes are used by nearly all sensory systems including vision, audition, somato-sensation, and olfaction and are thought to be advantageous for distinguishing between similar stimuli and for learning associations. In dense codes, the timing of spikes has been shown to contain sensory information, but the role of timing in the relatively few spikes in sparse sensory codes is unclear.
We used the olfactory system of awake locusts to test whether the timing of spikes in Kenyon cells, a population of neurons that respond sparsely to odors, carries sensory information to, and influences, follower neurons. First, we characterized two major classes of direct followers of Kenyon cells in a brain area called the β-lobe. With paired intracellular and field-potential recordings made during odor presentations, we found that the followers portray odor identity in the temporal patterns of their spikes but not in the spike rate, the spike phase, or the identities of active neurons. We also found that subtly manipulating the relative timing of Kenyon cell spikes, with temporally and spatially structured electrical microstimulation, reliably altered the responses of the followers. Our results show that even the remarkably sparse spiking responses of Kenyon cells provide information through odor-specific variations in timing on the order of tens to hundreds of milliseconds and that these variations determine responses downstream.
Sparse coding in sensory areas has been viewed as an outcome of, rather than as a substrate for, temporal processing. Stimulus-specific variations in spike timing provide a useful channel to increase the coding capacity of neurons while retaining the benefits of sparseness. Our results establish the importance of spike timing in sparse sensory codes.
Neural codes for odors in a deeper brain area
An important goal of our laboratory is to understand how sensory information moves from point to point through the nervous system, from more peripheral to deeper regions. In the locust, olfactory information is sent from peripheral olfactory organs to the antennal lobe. There, information is synchronized across cells into approximately 20 Hz oscillations and is then carried by projection neurons to two higher olfactory centers. The first center, the mushroom body, has been studied extensively and is thought to be a site for olfactory learning. However, the second center, the lateral horn (LH), remains poorly understood. It has been proposed that this area of the insect brain plays several important roles in odor coding, including maintaining the sparseness of olfactory responses by means of feed-forward inhibition and mediating behaviors elicited by innately meaningful odors. Yet, relatively little is known of the structure and function of LH neurons (LHNs), making it difficult to evaluate these ideas.
We surveyed more than 250 LHNs in locusts, using intracellular recordings to characterize their responses to sensory stimuli, dye-fills to characterize their morphologies, and immunostaining to characterize their neurotransmitters. We found a great diversity of LHNs, suggesting that this area may play several roles. Surprisingly, however, we found no evidence to support a role for these neurons in the feed-forward inhibition that has been proposed to mediate olfactory response sparsening; instead, it appears that another mechanism, feed-back inhibition from the giant GABAergic neuron (GGN), serves this function. Further, all LHNs we observed responded to all tested odors, making it unlikely that the LHNs could mediate specific behavioral responses to specific odors. Our results rather point to three other possible roles of LHNs: extraction of general stimulus features such as odor intensity; bilateral integration of sensory information; and integration of multimodal sensory stimuli.
Information capacity in olfactory neurons exhibiting oscillatory synchrony
Understanding how information is transmitted within the nervous system is essential to developing targeted therapies for neurological disorders. An important aspect of information transmission is the way information is represented by the electrical activity of neurons. One formatting strategy throughout the brain is the coordinated firing of groups of neurons through synchronous oscillations. Many benefits of synchrony for neural processing have been proposed. However, synchrony may have adverse consequences for coding. Given that the periodic inhibition underlying synchrony reduces the number of patterns any single neuron can produce, this strategy may ‘buy’ the benefits of synchronization at some cost to the brain’s ability to represent the external environment. Alternatively, inhibition could specifically remove noisy, low-information spikes, leading to only small changes in information following removal of inhibition.
We evaluated this fundamental tradeoff between coding format and information capacity in the olfactory system of the locust. To make our stimulation as relevant as possible, we developed a novel stimulus paradigm that matched the statistical structure of the animal’s environment. We made simultaneous recordings from groups of neurons to repeated presentations of this stimulus, both in control conditions and following delivery of the GABAa antagonist picrotoxin. To assess the capacity of the system to encode the stimulus, we measured the mutual information rate before and after picrotoxin application. We chose mutual information, as it quantifies how much can be known about the stimulus by observing the response. In recordings from individual neurons and simultaneously recorded pairs of neurons, we found that blocking synchronization led to modest increases in information rates (about 10–25%). We found that information efficiency (info/spike) was unchanged following desynchronization, while firing rates increased. We interpret the results to indicate that the transformation to a synchronous coding scheme via inhibition modestly reduces the ability of the olfactory system to transmit information about environmental stimuli. The study is the first to measure information-theoretic quantities while experimentally altering the correlation structure of neurons.
Information processing in the gustatory system
The gustatory system detects a bewildering array of chemicals. One influential theory posits that, in animals as diverse as flies and humans, gustatory systems recognize only small numbers of basic tastes such as sweet, salty, sour, bitter, and umami. We tested this in the gustatory system of an insect, the moth Manduca sexta, which has a relatively simple nervous system. Using a novel tastant-delivery system, we gave carefully timed stimuli to awake moths, while making intracellular and extracellular recordings from gustatory receptor neurons (GRNs).
We found that some GRNs responded to only one of the 16 diverse tastants that we tested, while other GRNs responded to many different tastants; thus, individual GRNs reported taste ambiguously. However, examining the responses of a population of GRNs together, we could reliably classify tastant identity and concentration, and even discriminate between chemicals from the same basic taste category, such as several different types of sugars or salts. A single GRN could be excited by some tastants, inhibited by others, or fire in complicated but reliable patterns. These firing patterns might be used to distinguish the responses of one tastant from another.
To determine how this information is processed in the moth’s brain, we made paired recordings from GRNs and their postsynaptic follower neurons to test their responses to tastants and to fill them with fluorescent dye for anatomical reconstruction. We found that GRNs synapse onto at least two general types of cells: projection neurons (ascending projection neurons that send axons to deeper brain regions, and descending projection neurons that send axons to motor centers innervating the insect’s legs, wings, and gut); and local neurons, some of which stained positively for the inhibitory neurotransmitter GABA. The follower neurons responded, with tastant-specific timing, to more tastants than their presynaptic GRNs; thus, multiple types of GRNs converge upon individual followers. We found that this gustatory system does not encode a small number of taste categories, but rather uniquely represents individual tastants using a distributed, spatiotemporal code. Our work sheds light on systems-level phenomena underlying the perhaps least understood of the five senses.
Spontaneous odor-receptor neuron activity determines follower cell response properties.
Many neurons and neural circuits display spontaneous activity. We are interested in how this activity arises, how it affects the function of the circuitry, and how it is propagated and controlled through successive levels of neural circuits. The insect olfactory system provides many advantages for investigating such fundamental questions.
In the olfactory system, the odor-receptor neurons in the antenna and their followers, second-order projection neurons and local neurons of the antennal lobe, all exhibit high levels of baseline activity in the absence of deliberately delivered stimuli. However, third-order neurons (Kenyon cells) exhibit very low baseline activity under the same conditions. (In the vertebrate olfactory system, similar observations have been made in first- and second-order neurons.) We used the locust olfactory system to explore where baseline activity originates and how it propagates through multiple layers of neurons.
We performed our experiments in intact animals. To locate the source of baseline activity, we reversibly silenced the receptor neurons by delivering chilled air to the antenna while using tetrode, intracellular, and patch recording techniques to track activity in each type of downstream cell. Cooling the antenna significantly lowered the spontaneous firing rate of the receptor neurons and had three main effects on downstream neurons. First, responses to odors were gradually eliminated. Second, the spontaneous firing rates of projection neurons decreased from 3–4 spikes/sec to less than 1 spike/sec. Finally, as the antenna cooled to about 6oC, the resting membrane potential of projection neurons fell by 6.9 mV, that of local neurons by 13.1mV, and that of Kenyon cells by 7.4 mV. The results demonstrate that the odor-receptor neurons provide a constant barrage of spontaneous input to later stages of olfactory processing. The input contributes to the spike rates of projection neurons and helps determine the resting membrane potentials of higher-order neurons.
How does the spontaneous activity arise? We found that baseline activity in the odor-receptor neurons arises within the sensilla, and not, for example, as a consequence of any residual, uncontrolled stimulation from our olfactometer. Baseline activity in the projection neurons was only marginally reduced by sealing the antenna in an odor-impermeable barrier. However, manipulations expected to rapidly clear odorants from the space extracellular to odor receptor neurons (by placing the antenna in a stream of rapidly moving air or by enveloping it in highly purified bottled air) significantly reduced baseline activity in projection neurons. The results suggest that noisy baseline activity originates from molecules lingering in the sensillar lymph surrounding the receptor neurons. Such baseline activity may be fundamentally unavoidable in a sensory neuron specialized to have a low threshold for detecting a variety of odorant molecules.
Given that a single odorant may activate multiple types of odor receptor neuron, that these neurons generate an ongoing barrage of noisy output, that each projection neuron receives input from relatively few types of receptor neuron, and that Kenyon cells receive massively convergent input from many projection neurons, the questions arises as to how the response thresholds of successive layers of follower neurons (projection neurons and Kenyon cells) are best set. With a simple receiver-operator model, we demonstrate that distinguishing signal from noise is best when projection neuron activity is minimally thresholded before the Kenyon cells, a configuration that permits the maximal convergence of information before a response decision is made. Taken together, our results illustrate how noise arises in a sensory system, how it propagates, and how it is controlled to provide effective signal detection.
Behavioral and physiological development of olfactory processing
How do the sensory capacities of animals develop? Animals often demonstrate, through their innate sensory preferences, the existence of inborn information. We are exploring how this information is encoded and how it differs from information acquired through direct experience.
We found that newly hatched locusts, when first crawling out of their hatching cups, immediately move toward fresh grass. In a series of behavioral studies using thousands of locusts, we established that the hatchlings choose: real grass rather than visually similar but odorless plastic grass; paper rubbed with fresh grass rather than clean paper of the same color; and paper dabbed with colorless monomolecular odorants that are components of grass odor (i.e., hexanol) rather than paper with other colorless odorants (even when the odorants had been mutually diluted to have identical vapor pressures). The results indicate that naive locusts, which have never eaten, touched, or otherwise encountered their natural food source, have a built-in preference for its odor.
The preference could be attributed to a peripheral mechanism; for example, perhaps hatchling locusts have a surplus of odor receptors for grass odors. We tested the hypothesis by making electro-antennograms from hatchlings and found that the antennae respond best to grass odor, and less strongly to an assortment of non-plant odors (as do, as we found, the antennae of adult locusts). This was also true when the odors were mutually diluted to provide equal vapor pressure. We also found that, in hatchling antennae, sensory adaptation occurs for grass odors with the same timing and extent as for non-plant odors. The results suggest that the behaviorally demonstrated innate preference for grass odors is determined, at least in part, by the prevalence of olfactory receptors for them, a prevalence that is in place at hatching and persists into adulthood.
In most respects, odor responses of the hatchling brain resemble those of the adult brain. By recording local field potentials in the mushroom bodies of hatchlings, we found that the oscillatory synchronization mechanism is already intact (although the oscillation frequency is significantly slower than in the adult). By recording intracellularly from antennal lobe neurons, we found odor-specific temporal patterns in the distributed responses of projection neurons. The responses appeared similar to those of adults, and we found no evidence in the antennal lobe for specialized neurons responding only to grass odors. Thus, representations of grass and other odors in the hatchling brain, as in the adult brain, are broadly, spatio-temporally distributed.
Additional Funding
- Additional funding from JSPS to Takaaki Miyazaki and Kazumichi Shimizu
Publications
- Assisi C, Stopfer M, Bazhenov M. Excitatory local interneurons enhance tuning of sensory information. PLoS Comput Biol 2012;8(7):e1002563.
- Gupta N, Stopfer M. Functional analysis of a higher olfactory center, the lateral horn. J Neurosci 2012;32:8138-8148.
- Joseph J, Dunn FA, Stopfer M. Spontaneous olfactory receptor neuron activity determines follower cell response properties. J Neurosci 2012;32:2900-2910.
- Tanaka N, Suzuki E, Dye L, Ejima A, Stopfer M. Dye-fills reveal additional olfactory tracts in the protocerebrum of wild-type Drosophila. J Comp Neurol 2012;520:4131-4140.
- Ong RC-Y, Stopfer M. Peripheral and central olfactory tuning in a moth. Chem Senses 2012;37:455–461.
Collaborators
- Maxim Bazhenov, PhD, Howard Hughes Medical Institute, The Salk Institute for Biological Studies, La Jolla, CA
- Kei Ito, PhD, University of Tokyo, Tokyo, Japan
- Chi-Hon Lee, MD, PhD, Program in Cellular Regulation and Metabolism, NICHD, Bethesda, MD
Contact
For more information, email stopferm@mail.nih.gov or visit neuroscience.nih.gov/Faculty/Profile/mark-stopfer.aspx.