Biophysics of Large Membrane Channels
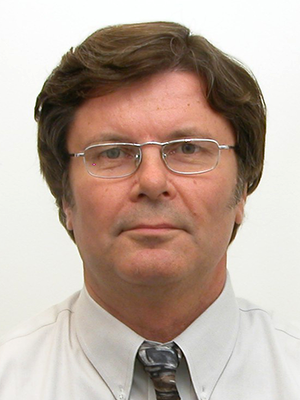
- Sergey M. Bezrukov, PhD, DSci, Head, Section on Molecular Transport
- Tatiana K. Rostovtseva, PhD, Associate Scientist
- Motahareh Ghahari Larimi, PhD, Visiting Fellow
- Megha Rajendran, PhD, Visiting Fellow
- Alexander M. Berezhkovskii, PhD, Contractor
- Kaitlin Abrantes, BS, Intramural Research Training Award Fellow
We study membrane transport through mitochondrial and bacterial membrane proteins that form “large” beta-barrel channels. These channels, recognized as novel drug targets, are the gateways for metabolite exchange as well as the components of many toxins. Healthy cell functioning and development require effective communication between cells and cell organelles, which is facilitated by membrane channels. Our motivation is that learning the molecular mechanisms of channel operation is vital for developing new approaches to treat various diseases in which regulation of transport through ion channels plays a key role. The benchmark approach of our lab is channel reconstitution into planar lipid membranes, a technique that we complement with the physical theory of transport and different biophysical and cell biology methods. This allows us to develop both qualitative and quantitative understanding of the transport processes. Currently, we mostly focus on the VDAC (voltage-dependent anion channel from the outer membrane of mitochondria), but also on other beta-barrel channels such as MspA (major outer-membrane porin from Mycobacterium smegmatis), alpha-Hemolysin (toxin from Staphylococcus aureus), OmpF (general bacterial porin from Escherichia coli), LamB (sugar-specific bacterial porin from Escherichia coli), OprF (porin from Pseudomonas aeruginosa), translocation pores of B. anthracis (PA63); C. botulinum (C2IIa), and C. perfringens (Ib) binary toxins are also within the range of our interest. Though these large channels are responsible for drastically different biological functions, they have similar beta-barrel structural motifs and biophysical properties, so that their comparative analysis provides an in-depth understanding of the underlying transport mechanisms.
The channel-reconstitution technique allows us to investigate large beta-barrel channels under precisely controlled conditions and to study transport at the single-molecule level. We first isolate the corresponding channel-forming proteins from their host organisms (or refold recombinant proteins from inclusion bodies), purify them, and then insert them into planar lipid membranes for electrophysiological examination. Our main goal is to elucidate the physical principles of regulation of such channels under normal and pathological conditions. In our latest projects, we supplement our biophysical methods with imaging techniques to study the role of the VDAC in mitochondria during embryonic development. Given the location of the VDAC at the outer mitochondrial membrane, we also use the Seahorse real-time metabolic assay platform to study its role in mitochondrial function. Among other wet-lab methods, we take advantage of fluorescence correlation spectroscopy, bilayer overtone analysis, and confocal microscopy. Results, obtained through this rich combination of refined biophysical techniques with live-cell imaging and other cell-biology techniques, inspire new strategies and pharmacological approaches to effectively correct the deviant molecular interactions associated with pathology in disease and development.
Regulation of mitochondrial respiration by membrane-bound peripheral proteins with disordered polyanionic C-terminal domains
The crucial role of mitochondrial outer membrane (MOM) permeability in maintaining an efficient metabolite exchange between mitochondria and cytoplasm in normal respiration is well established, with the VDAC recognized as the key metabolite pathway and regulator in MOM. It is believed that the uniqueness of this relatively simple monomeric beta-barrel channel mainly arises from its crucial position at the interface between a mitochondrion and the cytosol. When reconstituted into lipid membranes, the VDAC responds to sufficiently large transmembrane potentials by transitioning to gated states in which ATP/ADP flux is reduced and calcium flux is increased. However, a major reason for uncertainty regarding the physiological role of the VDAC voltage gating for the regulation of MOM permeability is the source and magnitude of the outer membrane potential in vivo.
We found that two otherwise unrelated cytosolic proteins, tubulin, and alpha-synuclein (α-Syn), dock with the VDAC by a newly established mechanism, in which the transmembrane potential draws their disordered, polyanionic C-terminal domains into and through the VDAC channel, thus physically blocking the pore. Remarkably, for both tubulin and α-Syn, the blocked states are observed at much lower transmembrane potentials than VDAC–gated states with their bulk concentrations as small as 10 nanomoles. Therefore, in the presence of these cytosolic docking proteins, the VDAC’s sensitivity to transmembrane potential is dramatically increased. The features of the VDAC voltage-gated states relevant for bioenergetics (reduced metabolite flux and increased calcium flux) are preserved in the blocked states induced by either docking protein. The ability of tubulin and α-Syn to modulate mitochondrial potential and ATP production in vivo is now supported by many studies. The common physical origin of the interactions of both tubulin and α-Syn with the VDAC leads to a general model of a VDAC inhibitor, facilitates predictions of the effect of post-translational modifications of known inhibitors, and, because of its generality, points the way toward the development of novel therapeutics targeting the VDAC.
Figure 1. Increased sensitivity of VDAC (PDB ID: 3EMN) regulation to transmembrane potential in the presence of cytosolic regulators
Only a fraction of the outer membrane potential, relative to that necessary to induce channel voltage gating (blue right-most curve), is required for the effective control of VDAC–induced transport by tubulin (red left-most curve) and alpha-Syn (green curve in the center) at their bulk concentrations as small as 10 nanomolar. The changes in the transport properties of the channel in response to the outer membrane potential, i.e., decrease in metabolite flux and the concomitant increase in calcium flux, remain qualitatively the same in all three cases. The “effective gating charge,” expressed as the steepness of the voltage-dependence curve, is the lowest for voltage gating and the highest for tubulin, with α-Syn in between.
Trapping of single diffusing particles by a circular disk on a reflecting flat surface
Recent progress in cell biophysics (for example, in studies of chemical sensing and spatiotemporal cell signaling) poses new challenges to the statistical theory of trapping of single diffusing particles. We offered an analytical solution to one of them, namely, the trapping kinetics of single particles diffusing in a half-space bounded by a reflecting flat surface containing an absorbing circular disk. Our analysis is quite general and is thus relevant for various applications involving not only signal transduction problems in biology but operation of different kinds of chemical sensors. Signaling within and between cells is the key phenomenon underlying the development and functional behavior of cells and multicellular organisms. The so-called “spatiotemporal dynamic cell-signaling modeling” focuses on signaling processes both in individual cells and the spatial coupling between different cells within a tissue. As follows from the name, the efficiency and characteristic duration of signal transduction between cells are studied as functions of their relative spatial location. Researchers point out that the response strength could depend on a vector distance between the signal origin and the receptor, rather than a scalar distance alone, thus exhibiting directional behavior. This trapping problem is essentially two-dimensional, and the question of the angular dependence of the kinetics on the particle starting point is highly nontrivial. We proposed an approximate approach to the problem that replaces the absorbing disk with an absorbing hemisphere of a properly chosen radius. The replacement makes the problem angular-independent and essentially one-dimensional. After the replacement, one can find an exact solution for the particle propagator (Green’s function) that allows one to completely characterize the kinetics. Extensive testing of the theoretical predictions based on the absorbing hemisphere approximation against three-dimensional Brownian dynamics simulations shows excellent agreement between the analytical and simulation results when the particle starts sufficiently far from the disk. It works well for distances of the particle starting point from the disk center comparable with the disk diameter or larger. This condition reflects actual situations in multiple signaling pathways at the cell and organism levels.
Figure 2. Brownian dynamics simulation cell
(A) Notations: r0 is the particle injection coordinate; Rout and a are radii of the absorbing sphere and disc on the surface.
(B) Results of simulations of splitting probability of the particle capture by the disk as a function of distance r0 at different starting angles relative to the surface (triangles: starting point at 900; circles: at 300; and squares: starting on the reflecting surface, 00 ) vs. theory (lines) of the sphere approximation.
(C) Corresponding mean first-passage times vs. theory.
Analytical theory for the permeability and diffusion resistance of porous membranes and its numerical test
Channels of cell and organelle membranes are often clustered, forming domains of tightly packed transport proteins. A well known example is the VDAC, the major gateway for metabolites and ions in the outer mitochondrial membrane. Indeed, electron micrographs of MOM fragments from Neurospora crassa and high-resolution atomic force imaging of MOM fragments from Saccharomyces cerevisiae show that, in both cases, VDAC beta-barrels form dense arrays of hundreds of channels. The characteristic distances between barrel centers are as small as 4 to 5 nm, which are comparable to the barrel outer diameter of about 3 nm. Estimates demonstrate that in these high-density domains, the VDAC protein occupies about 80% of the membrane surface. Previously, we considered the effect of clustering on particle trapping by absorbing disks and channel-facilitated transport, assuming that the size of the cluster is much smaller than the total membrane area. This year, we addressed a different problem. Specifically, we studied the case in which the channels are spread over the entire membrane, with a focus on the effects of channel “crowding” on membrane transport. We considered the transport of neutral solutes through porous flat membranes, driven by the solute concentration difference in the reservoirs separated by the membrane. Transport occurs through membrane channels, which are assumed to be non-overlapping, identical, straight cylindrical pores connecting the reservoirs. The key quantities characterizing transport are membrane permeability and diffusion resistance. Such transport problems, arising in very different contexts ranging from plant physiology and cell biology to chemical engineering, have been studied for more than a century. Nevertheless, an expression giving the permeability for a membrane of arbitrary thickness at arbitrary surface densities of the channel openings has been unknown. We have filled this gap and derived such an expression. Given that this expression involves approximations, we compared its predictions with the results obtained from Brownian dynamics simulations and found excellent agreement between the two.
Publications
- Rostovtseva TK, Bezrukov SM, Hoogerheide DP. Regulation of mitochondrial respiration by VDAC is enhanced by membrane-bound inhibitors with disordered polyanionic C-terminal domains. Int J Mol Sci 2021 22:7358.
- Dagdug L, Berezhkovskii AM, Zitserman VY, Bezrukov SM. Effective diffusivity of a Brownian particle in a two-dimensional periodic channel of abruptly alternating width. Phy Rev E 2021 103:062106.
- Skvortsov AT, Dagdug L, Berezhkovskii AM, Bezrukov SM. Blockage coefficient of cylindrical blocker and diffusion resistance of membrane channels. Phys Fluids 2023 35:011702.
- Dagdug L, Berezhkovskii AM, Bezrukov SM. Trapping of single diffusing particles by a circular disk on a reflecting flat surface. Absorbing hemisphere approximation. Phys Chem Chem Phys 2023 25:2035–2042.
- Skvortsov AT, Dagdug L, Hilder EF, Berezhkovskii AM, Bezrukov SM. Permeability and diffusion resistance of porous membranes: Analytical theory and its numerical test. J Chem Phys 2023 158:054114.
Collaborators
- Vicente M. Aguilella, PhD, Universidad Jaume I, Castellón, Spain
- Mark R. Cookson, PhD, Laboratory of Neurogenetics, NIA, Bethesda, MD
- Leonardo Dagdug, PhD, Universidad Autónoma Metropolitana-Iztapalapa, Mexico City, Mexico
- David Hoogerheide, PhD, National Institute of Standards and Technology, Gaithersburg, MD
- Jennifer C. Lee, PhD, Biochemistry and Biophysics Center, NHLBI, Bethesda, MD
- Ekaterina M. Nestorovich, PhD, The Catholic University of America, Washington, DC
- Olga Protchenko, PhD, Liver Diseases Branch, NIDDK, Bethesda, MD
- Alexander Sodt, PhD, Unit on Membrane Chemical Physics, NICHD, Bethesda, MD
- Gerhard Wagner, PhD, Harvard Medical School, Cambridge, MA
- Michael Weinrich, MD, Office of the Director, NICHD, Bethesda, MD
- David L. Worcester, PhD, National Institute of Standards and Technology, Gaithersburg, MD
- Joshua Zimmerberg, MD, PhD, Section on Integrative Biophysics, NICHD, Bethesda, MD
Contact
For more information, email bezrukos@mail.nih.gov or visit https://www.nichd.nih.gov/research/atNICHD/Investigators/Bezrukov.