Neuregulin–ErbB and NMDA Receptor Signaling in Neuronal Development and Psychiatric Disorders
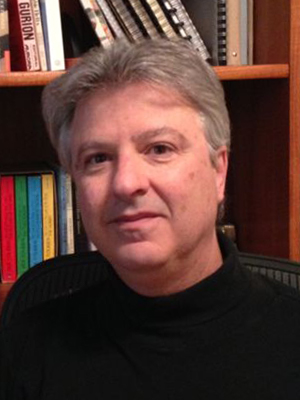
- Andres Buonanno, PhD, Head, Section on Molecular Neurobiology
- Detlef Vullhorst, PhD, Staff Scientist
- Eastman Lewis, PhD, Postdoctoral Intramural Research Training Award Fellow
- Ricardo Murphy, BS, Contractor
Failure of cortical microcircuits to properly regulate excitatory-inhibitory (E-I) balance is a key feature in the etiology of several developmental psychiatric disorders and neurological diseases, such as schizophrenia, autism, ADHD, and epilepsy. E-I balance is important to synchronize the firing pattern of local neuron ensembles, and its dysregulation can degrade cognitive functions and, in extreme cases, result in epileptiform activity. Alterations in neuronal network activity, in particular oscillations in the gamma-frequency range (30–80 Hz), are associated with behavioral and cognitive deficits in psychiatric disorders. We have been investigating whether and how Neuregulins (NRG 1-3) and their major neuronal receptor ErbB4, which are genetically linked to psychiatric disorders, function in an activity-dependent fashion (i.e., experience) in the developing brain to regulate synaptic and neuronal network properties. We use genetically modified NRG and ErbB4 mouse models, in combination with optogenetic, electrophysiological, behavioral, and molecular/cellular techniques to identify novel interactions between the NRG/ErbB4, glutamatergic, dopaminergic, and GABAergic signaling pathways associated with psychiatric disorders.
Our earlier studies demonstrated that NRG/ErbB4 signaling in GABAergic fast-spiking parvalbumin-positive (PV+) interneurons regulates E-I balance, gamma oscillation network activity, and numerous behaviors relevant to psychiatric disorders. To understand how NRGs mediate their biological functions during brain development, we investigated how different NRG ligands are proteolytically processed and trafficked in neurons, using molecular, cellular, and genetic approaches. NRGs are synthesized as unprocessed pro-proteins (proNRGs) containing either a single or two transmembrane (TM) domains, and, contrary to dogma, we discovered that these two types of NRGs are processed and trafficked very differently. Single–TM NRGs are cleaved and shed from endoplasmic reticulum–plasma membrane (ER–PM) junctions on neuronal soma in an activity-dependent fashion in response to glutamate NMDA receptor (NMDAR) activation. By contrast, dual-pass TM NRGs are constitutively cleaved by BACE1 in the trans-Golgi network, sorted into axons by transcytosis, and then selectively retained at presynaptic glutamatergic terminals via juxtacrine trans-synaptic interactions with ErbB4 receptors on dendrites of GABAergic interneurons (Figure 1). These findings suggest that single-pass TM NRGs signal in paracrine mode at neuronal soma and proximal dendrites, whereas dual-pass TM NRGs signal in juxtacrine fashion from axons and presynaptic terminals by interacting with postsynaptic ErbB4 on GABAergic dendrites.
Figure 1. Distinct subcellular distribution of NRG2 and NRG3 in a cultured hippocampal ErbB4+ GABAergic interneuron
A dissociated hippocampal GABAergic interneuron showing pro–NRG2 surface puncta (white), clustered at ER–PM contacts, and processed NRG3 at presynaptic terminals (green) that are juxtaposed to postsynaptic ErbB4 receptors (red). Note the restricted distribution of pro–NRG2 on the soma and proximal dendrites that correspond to clusters of NRG2 at ER–PM junctions, as confirmed by electron microscopy (inset). In stark contrast to NRG2, NRG3 and ErbB4 staining overlap extensively, consistent with their trans-synaptic interactions at excitatory synapses onto inhibitory interneurons (see also Figure 2).
We are interested in how NRG/ErbB4 signaling would affect networks and behaviors associated with psychiatric disorders. The prefrontal cortex (PFC) is a site of convergence of long-range glutamatergic inputs, which integrates multiple modalities of information to produce goal-directed behaviors. GABAergic PV+ interneurons coordinate pyramidal cell firing induced by this converging glutamatergic innervation to synchronize cortical networks that modulate goal-oriented behaviors, which are disrupted in psychiatric disorders. Interestingly, drugs that inhibit NMDAR activity (i.e., ketamine) alter E-I balance, increase gamma oscillation network activity, and disrupt behaviors associated with psychiatric disorders. Several lines of evidence suggest that NMDAR antagonists may disproportionally act on NMDARs expressed on PV+ interneurons; however, there has been significant controversy as to whether adult PV+ interneurons have functional NMDARs. Given the importance of PV+ interneurons for cortical functions, and our prior findings that NRG/ErbB4 signaling inhibits NMDAR activity in GABAergic interneurons, we embarked on an investigation of the proportion of PFC adult PV+ interneurons expressing functional NMDARs and their role in local network activity.
Presynaptic accumulation of NRG3 in central neurons is achieved by trans-synaptic retention: a novel mechanism for polarized axonal expression of proteins.
How stable axonal polarity is maintained remains a central question in neuroscience. We previously demonstrated that dual–TM proNRGs, comprising CRD-NRG1 type III and NRG3, are targeted to axons and accumulate at glutamatergic presynaptic terminals, where they signal in juxtacrine mode via postsynaptic ErbB4 receptors expressed at postsynaptic densities on GABAergic interneurons [Vullhorst, Ahmad et al. J Neurosci 2017; Figure 1]. Our new study aimed to understand how and where proNRG3 is cleaved by BACE1 and how the resulting biologically active NRG3 peptide is sorted and selectively retained in axons. For this study, an LOV (light-oxygen-voltage)–based optogenetic proNRG3 reporter (LA143–NRG3) was designed, which, in response to blue light, undergoes a conformational change that allows BACE1 to cleave proNRG3. Using LA143–NRG3, we found that proNRG3 is retained in the TGN until cleaved by BACE1, whereupon mature NRG3 emerges on the somatodendritic plasma membrane and, by transcytosis, is re-endocytosed and anterogradely transported on Rab4+ (small GTPases involved in intracellular vesicle trafficking) vesicles into axons. Importantly, NRG3 accumulation at axonal presynaptic terminals is maintained by its continued trans-synaptic interaction with ErbB4 receptors expressed at postsynaptic glutamatergic synapses on GABAergic interneurons (Figure 2). We denote this novel mechanism “trans-synaptic retention” and propose that it can account for polarized axonal expression of other transmembrane ligands and receptors [Reference 1].
Figure 2. Distinct subcellular distribution of NRG2 and NRG3 in a cultured hippocampal ErbB4+ GABAergic interneuron
The diagram depicts the different steps of pro–NRG3 processing, which begin with its synthesis as a dual–TM protein in the endoplasmic reticulum, its cleavage, and sorting of the biologically active single–TM NRG3 ligand to axonal presynaptic terminals, where it is retained by virtue of its interaction with postsynaptic ErbB4 receptors. The three major steps of pro–NRG3 processing and axonal retention are:
- Unprocessed dual–TM pro-NRG3 requires cleavage by the protease BACE1 to release the biologically active single–TM NRG3 from the trans-Golgi network (TGN).
- NRG3 then traffics to axons by transcytosis, a process that involves initial transport of NRG3 to the somatodendritic plasma membrane, re-endocytosis into Rab5–positive early endosomes, sorting, and anterograde axonal transport in Rab4–positive vesicles.
- NRG3 is retained selectively at axonal terminals by virtue of its binding across the synapse to its cognate receptor ErbB4, which is expressed at glutamatergic postsynaptic densities on dendrites of GABAergic interneurons. We termed this mechanism, which is necessary for the polarized expression of NRG3 in axonal terminals, "trans-synaptic retention," and we propose that it accounts for the polarized expression of other neuronal transmembrane ligands and receptors in axons.
ER–PM junctions on GABAergic interneurons are organized by neuregulin 2/VAP interactions and regulated by NMDA receptors.
Two major unresolved questions we recently pursued were to understand, at a mechanistic level: (1) how proNRG2 clusters at ER–PM junctions; and (2) how proNRG2 dissociates from the junctions in response to NMDAR activation. We found that proNRG2 promotes the formation of ER–PM junctions in hippocampal GABAergic interneurons via interactions of its cytoplasmic tail with the ER–resident protein VAP. Interestingly, there are two stretches of amino acids in the intracellular cytoplasmic domain conserved between proNRG1 and proNRG2, denoted C- and D-boxes, which are required to stabilize proNRG2/VAP complexes during immunoprecipitation. Although the protein sequence of neither box conforms to known FFAT (acronym for two phenylalanines in an acidic tract) motifs, shown in other proteins to bind to VAP, the proNRG2 D-box contains a track of acidic residues required for VAP binding and the C-box harbors a cryptic, phosphorylation-dependent VAP–binding site. Importantly, NMDAR activation stimulates dephosphorylation of Ser/Thr residues in the C-box and its dissociation from VAP, which reduces proNRG2 clustering at ER–PM junctions [Reference 2]. These observations are interesting because, although both proNRG2 and the potassium channel Kv2.1 are colocalized at ER–PM junctions and clustering at these sites is regulated by NMDA receptor activity, their modes of interaction with VAP differ (Figure 3). Based on these findings, we hypothesize that autocrine NRG2/ErbB4 signaling and Kv2.1 function synergistically as a homeostatic protective mechanism to downregulate GABAergic interneuron excitability during periods of strong excitatory activity and/or elevated extracellular glutamate levels, which would help protect these neurons from excitotoxicity.
Figure 3. ProNRG2 clustering at ER–PM junctions is mediated by VAP binding and regulated by phosphorylation.
A. Cultured hippocampal neurons transduced with AAVs expressing wild-type (WT) proNRG2 (top) or proNRG2DCDD lacking the C- and D-boxes (bottom). WT NRG2 (green) forms large clusters at ER–PM junctions (revealed by Kv2.1 staining in red), which are located on the cell body and proximal dendrites. Removal of the VAP binding sites in proNRG2DCDD abolishes its accumulation at ER–PM junctions, causing it to broadly distribute throughout the neuronal plasma membrane.
B. Working model of proNRG2/VAP interactions. ProNRG2 cooperatively engages with a VAP dimer via its two low-affinity FFAT motifs in the C- and D-boxes. Interactions between the VAP major sperm protein (MSP) domain and the cryptic FFAT site in the C-box require Ser/Thr phosphorylation. Their dephosphorylation downstream of NMDAR activation promotes proNRG2 dissociation from VAP.
Single-pass TM NRG2 in central neurons
Single-pass TM NRGs, such as NRG1 type II and NRG2, traffic as unprocessed pro-forms to the neuronal cell surface, where they accumulate at ER–PM junctions on neuronal soma and proximal dendrites. Activation of excitatory glutamatergic NMDARs located on neuronal soma/dendrites promote calcium entry and activate phosphatases that dephosphorylate Ser/Thr residues in the proNRG2 intracellular region, resulting in the dissociation of proNRG2 from ER–PM junctions and ectodomain cleavage by the metalloproteinase ADAM10 [Vullhorst D, Buonanno A. Mol Neurobiol 2019;56:8345]. The released, biologically active NRG2 binds to and activates ErbB4 receptors expressed at postsynaptic excitatory glutamatergic synapses onto GABAergic interneurons, which in turn selectively downregulates NMDA excitatory currents [Vullhorst et al, Nat Comm 2015;6:7222]. We hypothesize that this bidirectional NMDAR–NRG2 (up)/ErbB4–NMDAR (down) signaling mode serves as a homeostatic mechanism that regulates the activity of GABAergic interneurons.
A remaining challenge was to understand how proNRG2 clusters at ER–PM junctions, a site where Kv2.1 potassium channels also accumulate via their interaction with VAP, and how NMDAR activation causes their dissociation from the junction. Using a combination of cell-biological and protein-biochemical approaches, we identified two conserved protein sequences (denoted C- and D-boxes) in the cytoplasmic tails of proNRG1 and proNRG2 that interact with the ER–resident protein VAP to promote the formation of ER–PM contact sites on the proximal somatodendritic region of GABAergic interneurons (Figure 3). Although the sequences of C/D-boxes do not conform to canonical FFAT VAP–binding sites, the proNRG2 D-box contains acidic residues required for VAP binding and the C-box harbors a cryptic, phosphorylation-dependent VAP binding site. Importantly, NMDAR activation stimulates C-box Ser/Thr dephosphorylation, dissociation of proNRG2 from VAP and dispersion of NRG2 from ER–PM junctions (Figure 3). Based on these findings, we hypothesize that Kv2.1 channels and bidirectional NRG2/ErbB4 autocrine signaling function synergistically as a homeostatic mechanism both to protect fast-spiking parvalbumin-positive interneurons from excitotoxic damage and to regulate the activity of GABAergic interneurons, which affect E-I balance and neuronal network activity associated with psychiatric-relevant behaviors disrupted in NRG2 and ErbB4 knockout mice [Reference 2].
Developmental, neurochemical, and behavioral analyses of ErbB4 Cyt-1 knockout mice
ErbB4 receptor transcripts are alternatively spliced to generate isoforms that either include (Cyt-1) or exclude (Cyt-2) exon 26, an exon that encodes a cytoplasmic domain that invests ErbB4 receptors with the ability to signal via the PI3K/Akt pathway rather than the MAPK pathway. To investigate the effects of germline (constitutive) and conditional (acute) deletions of the Cyt-1 exon, we generated and studied ErbB4–floxed (ErbB4-Cyt1fl/fl) mice because ErbB4 Cyt-1/2 isoforms had been only studied in cultured cells, and clinical studies implicated ErbB4 Cyt-1 variants as schizophrenia risk factors. We found Cyt-1 knockouts (KOs) only encode ErbB4 Cyt-2 variants, but in contrast to ErbB4 null mice, GABAergic interneuron migration and number are unaltered in Cyt-1 KOs. Interestingly, basal extracellular dopamine (DA) levels are augmented in the medial prefrontal cortex of Cyt-1 mice, but they don't manifest the behavioral abnormalities we observed in mice lacking ErbB4 in DA neurons. To address the possibility that Cyt-2 variants compensate for the lack of Cyt-1 during development, we microinjected AAV (adeno-associated virus)–Cre into the DA–rich VTA (ventral tegmental area) of adult ErbB4-Cyt1fl/fl mice to acutely target exon 26. These conditional Cyt-1 KOs were found to exhibit behavioral abnormalities in the elevated plus maze and startle response, consistent with the idea that late exon 26 ablations may circumvent compensation by Cyt-2 variants. Our findings suggest that ErbB4 Cyt-1 function in vivo is important for modulating DA levels and for regulating behaviors in adult mice [References 3,4].
Pathway-specific contribution of parvalbumin interneuron NMDARs to synaptic currents and thalamocortical feedforward inhibition
The PFC is a site of convergence of long-range excitatory glutamatergic inputs, which integrates multiple modalities of information to produce goal-directed behaviors, and drugs selectively targeting NMDA–type glutamate receptors alter PFC function and elicit numerous deficits associated with psychiatric disorders. Inhibitory GABAergic fast-spiking PV+ interneurons are uniquely suited to coordinate the firing of pyramidal neurons in response to these converging excitatory inputs, and to induce gamma oscillations in cortical networks that modulate behaviors and may be disrupted in several psychiatric disorders. Despite the importance of understanding how glutamatergic inputs onto PV+ interneurons affect network activity, behavior, and disease, when we began this project, there continued to be controversy as to whether both AMPA– and NMDA–type glutamate receptors or only AMPARs contribute to excitatory drive. We used a combination of molecular, electrophysiological, and optogenetic approaches, in combination with selective gene targeting techniques in PV+ interneurons, to resolve this long-standing controversy. We found that nearly 100% of PV+ interneurons in the adult medial PFC express transcripts encoding GluN1 and GluN2B, which assemble to form functional NMDARs. Importantly, by using selective optogenetic stimulation of corticocortical vs. thalamocortical inputs onto PV+ interneurons, we found that the relative synaptic NMDAR contribution to excitatory post-synaptic currents is pathway-specific. NMDARs contribute more at thalamocortical, rather than at corticocortical, synapses. We then demonstrated that PV+ interneuron NMDAR currents contribute to thalamus-mediated feedforward inhibition in PFC circuits (Figure 4). These findings, demonstrating a molecular and circuit-based mechanisms for cognitive impairment under conditions of reduced NMDAR function, represent an important conceptual advance, with implications for understanding the pathogenesis of psychiatric disorders [Reference 5].
Figure 4. Pathway-specific contribution of PV+ interneuron NMDARs to synaptic currents and thalamocortical feedforward inhibition
Inhibitory fast-spiking GABAergic PV+ interneurons (red) in adult mouse PFC receive excitatory glutamatergic inputs from the contralateral PFC (cPFC; orange) and the ipsilateral thalamus (green). Optogenetic stimulation of either cPFC or thalamocortical inputs onto PV+ interneurons indicate that NMDARs contribute to the size and shape of excitatory synaptic currents at thalamic more than at cPFC synapses. By selectively knocking out NMDAR expression in adult inhibitory PV+ interneurons, we found that expression of these receptors at thalamic synapses of PFC PV+ interneurons are required for feedforward inhibition of pyramidal cells (PC).
Members of the Section on Molecular Neurobiology
Section on Molecular Neurobiology members who contributed to the work described in this Annual Report. Left to right: Dr. Andres Buonanno (Section Head, Senior Scientist), Dr. Eastman Lewis (Research Fellow), Ms. Jacqueline Welday (PostBac), Ms. Hayli Spence-Osorio (PostBac), Dr. Detlef Vullhorst (Staff Scientist), Ms. Mara Bloom (PostBac) and Mr. Ricardo Murphy (Senior Animal Biologist).
Additional Funding
- NICHD DIR Director's Investigator Award (RRC# D-14-07). PI: Andres Buonanno; Co-PI: Juan Bonifacino. “Exploring the functional role of Neuregulin isoform diversity in CNS”
- Center for Compulsive Behaviors Fellowship Award
Publications
- Ahmad T, Vullhorst D, Chaudhuri R, Guardia CM, Chaudhary N, Karavanova I, Bonifacino JS, Buonanno A. Transcytosis and trans-synaptic retention by postsynaptic ErbB4 underlie axonal accumulation of NRG3. J Cell Biol 2022 221:e202110167.
- Vullhorst D, Bloom MS, Akella N, Buonanno A. ER-PM junctions on GABAergic interneurons are organized by neuregulin 2/VAP interactions and regulated by NMDA receptors. Int J Mol Sci 2023 24(3):2908.
- Erben L, Welday JP, Cronin ME, Murphy R, Skirzewski M, Vullhorst D, Carroll SL, Buonanno A. Developmental, neurochemical, and behavioral analyses of ErbB4 Cyt-1 knockout mice. J Neurochem 2022 161:435–452.
- Erben L, Welday JP, Murphy R, Buonanno A. Toxic and phenotypic effects of AAV_Cre used to transduce mesencephalic dopaminergic neurons. Int J Mol Sci 2022 23(16):9462.
- Lewis EM, Spence HE, Akella N, Buonanno A. Pathway-specific contribution of parvalbumin interneuron NMDARs to synaptic currents and thalamocortical feedforward inhibition. Mol Psychiatry 2022 27:5124–5134.
Collaborators
- Tanveer Ahmad, PhD, Multidisciplinary Centre for Advance Research and Studies, Jamia Millia Islamia, New Delhi, India
- Juan Bonifacino, PhD, Section on Intracellular Trafficking, NICHD, Bethesda, MD
- Steve Carroll, MD, PhD, Medical University of South Carolina, Charleston, SC
- Carlos Guardia, PhD, Section on Intracellular Trafficking, NICHD, Bethesda, MD
- Miguel Skirzewski, PhD, University of Western Ontario, London, Canada
- Jung-Hwa Tao-Cheng, PhD, EM Facility, NINDS, Bethesda, MD
Contact
For more information, email buonanno@mail.nih.gov or visit https://www.nichd.nih.gov/research/atNICHD/Investigators/buonanno.