Building the Zebrafish Lateral Line System
- Ajay Chitnis,
MBBS, PhD, Head, Section on Neural Developmental Dynamics - Gregory Palardy, BS, Research Technician
- Chongmin Wang, MS, Research Technician
- Pritesh Krishnakumar, PhD, Postdoctoral Fellow
- Sana Fatma, PhD, Postdoctoral Visiting Fellow
- Abhishek Mukherjee, PhD, Postdoctoral Visiting Fellow
- Seth Entriken, BSc, Postbaccalaureate Fellow
- Avaneesh Kilnagar, BSc, Postbaccalaureate Fellow
- Julia Rashid, BSc, Postbaccalaureate Fellow
- Arin Rinvelt, BA, Postbaccalaureate Fellow
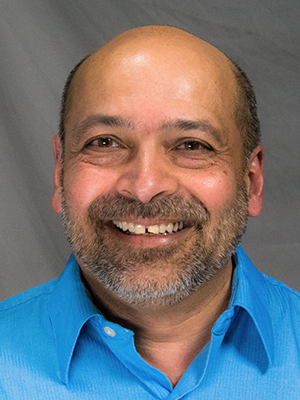
Cells divide, move, adhere, and interact with their neighbors and their environment to determine the formation of multicellular organ systems with unique fates, morphologies, function, and behavior. Our goal is to understand how such interactions determine the self-organization of cell communities in the nervous system of the zebrafish (Danio rerio) embryo. The lateral line is a mechano-sensory system that helps sense the pattern of water flow over the fish and amphibian body; it consists of sensory organs called neuromasts, which are distributed in a stereotypic pattern over the body surface. Each neuromast has sensory hair cells at its center, surrounded by support cells that serve as progenitors for the production of more hair cells during growth and for the regeneration of neuromasts. The development of this superficial sensory system in zebrafish is spearheaded by the posterior lateral line primordia (pLLp), groups of about 150 cells formed on either side of a day-old embryo near the ear. Cells in the primordia migrate collectively under the skin to the tip of the tail as they divide and reorganize to form nascent neuromasts, which are deposited sequentially from the primordium's trailing end. Their journey is easily observed in live transgenic embryos with fluorescent primordium cells. Furthermore, a range of genetic and cellular manipulations can be used to investigate gene function and morphogenesis in the system. Understanding the self-organization of this relatively simple and accessible system in zebrafish will help elucidate the broader principles that determine cell-fate specification, morphogenesis, and collective cell migration in the developing vertebrate nervous system.
Signaling and mechanics influence the number and size of epithelial rosettes in the migrating zebrafish posterior lateral line primordium.
Protoneuromasts are formed within the migrating primordium, starting from its trailing end, as clusters of cells apically constrict and form epithelial rosettes. Their formation is promoted by fibroblast growth factor (Fgf)–signaling centers, which form periodically in the wake of a shrinking Wnt–active domain that inhibits epithelial rosette formation and progressively shrinks toward the leading end of the primordium (Wnt and Fgf pathways are signaling pathways). However, the precise number and size of epithelial rosettes is not strictly dependent on a pre-pattern of Fgf–signaling activity, as it is broadly influenced by the balance of mechanical interactions that promote or oppose formation of epithelial rosettes. When chemokine-dependent migration of leading cells is compromised, the resulting slowing of the primordium is accompanied by the fusion of epithelial rosettes to form fewer larger rosettes. However, such fusion is not observed when Fgf signaling, responsible for migration of trailing cells, is inhibited to slow primordium migration. These observations can be accounted for by a mechanics-based model, in which local interactions associated with apical constriction and cell adhesion promote aggregation, while tension along the length of the primordium, influenced by the relative efficacy of leading and trailing cell migration, opposes such aggregation. We described the development of a computational Cellular Potts model, which allowed us to explore how the relative speed of leading versus trailing cells, as well as changes in cell adhesion and mechanical coupling, differentially regulated by Wnt and Fgf signaling, can influence the pattern of neuromast formation and deposition by the migrating primordium. Our studies illustrate how signaling and mechanics cooperate to coordinate self-organization of morphogenesis in the migrating primordium.
Sox2 helps stabilize neuromasts prior to deposition, defining a final key step in the self-organization of neuromast formation in the migrating posterior lateral line primordium.
Neuromasts, the mechanosensory organs of the lateral line system, are periodically formed and deposited by the migrating pLLp from its trailing end, as it migrates under the skin from the near the ear to the tip of the tail, to spearhead formation of the posterior lateral line system in zebrafish. Understanding how they are formed and deposited in a robust and reproducible way serves as a model for understanding more broadly the steps that determine the reproducible self-organization of organ systems during development.
The pLLp forms as a group of about 140 cells separates from an epithelial placode located behind the ear. Prior to the initiation of migration, cells in the primordium are characterized by broad, relatively uniform Wnt regulatory activity. However, as the primordium begins to migrate caudally towards a source of chemokine signals secreted by the horizontal myoseptum, Wnt activity becomes polarized such that it is highest at the leading end of the primordium and lowest at its trailing end. Such polarization provides the context for subsequent patterning events within the primordium that coordinate both polarized migration of the primordium along a relatively uniform stripe of chemokine expression and for the periodic formation and deposition of neuromasts.
Protoneuromast formation is initiated in Fgf–signaling domains that are periodically established in a trailing zone of the migrating primordium in response to Fgfs produced by Wnt–active cells in a leading zone. Local promotion of Wnt activity, coupled with longer range inhibition of Wnt by Fgf–dependent Dkk1b (a protein that inhibits Wnt) expression, is thought to constitute a reaction-diffusion system that determines self-organization of Fgf signaling centers, which initiate formation of nascent protoneuromasts. As previously formed protoneuromasts at the trailing end mature, they produce Wnt–signaling inhibiting factors, which contribute to progressive shrinking of the Wnt system. Progressive restriction of an initially broad Wnt–signaling domain to a smaller leading zone allows new Fgf signaling–dependent protoneuromasts to form in the wake of the shrinking Wnt system.
While initial studies focused on Dkk1b as the primary inhibitor of Wnt signaling produced by newly formed protoneuromasts, a question arose about what inhibits Wnt signaling in the most mature protoneuromasts at the trailing end of the primordium, where dkk1b is not expressed. We showed that Sox2 (a transcription factor that maintains pluripotency and neurogenesis) is expressed in nascent and maturing protoneuromasts in a pattern that is complementary to domains with Wnt signaling activity. Furthermore, Sox2 functions in a partially redundant manner with Sox1a and Sox3 to inhibit Wnt signaling. This helps keep Wnt activity restricted to a progressively smaller leading zone, which we show is essential for effective maturation of trailing protoneuromasts and the timely deposition of stable neuromasts.
Fgfs secreted by Wnt–active cells in a leading zone determine Fgf signaling activity in an adjacent trailing zone, which initiates protoneuromast formation by promoting expression of factors that promote epithelialization of cells and their apical constriction to form epithelial rosettes. Furthermore, Fgf signaling promotes expression of the transcription factor atoh1a, which gives cells the potential to become sensory hair-cell progenitors, while lateral inhibition mediated by Notch signaling, which inhibits atoh1a expression during neurogenesis, ensures center-biased atoh1a expression, and sensory hair-cell progenitor fate becomes restricted to a central cell in the nascent protoneuromast. However, as the protoneuromast matures in a trailing zone of the primordium, it becomes increasingly separated from leading Wnt–active cells, which are the source of Fgfs that initiated its formation. As a result, effective maturation requires a switch to a new regulatory network that ensures sustained Fgf activity in the maturing protoneuromast, independent of the Fgfs from the leading Wnt–active zone that initiated its formation. This is achieved by the central Atoh1a–expressing sensory hair-cell progenitor, which becomes a new source of Fgfs that sustain Fgf signaling in surrounding protoneuromast cells. In addition, the central Atoh1a–expressing cell determines expression of Notch ligands such as DeltaD, which activate Notch in neighboring cells to promote expression of factors that ensure stabilization of epithelial rosette morphology. Importantly, however, as the Atoh1a–expressing cell in the maturing proneuromast becomes separated from leading Wnt–active cells, which are the source of Fgfs that initiate atoh1a expression, Atoh1a promotes atoh1b expression and sustains its own expression through autoregulation.
Our analysis of Sox2 function has now shown that this critical switch in the genetic regulatory network, where Atoh1a expression becomes self-sustaining through autoregulation with atoh1b, only occurs when Wnt activity is adequately suppressed in trailing cells by Sox2. Failure to adequately suppress and restrict Wnt activity to a leading zone prevents timely initiation of atoh1b expression in trailing protoneuromasts and failure to deposit mature neuromasts with stable epithelial rosettes. Together with past observations, our analysis of Sox2 function now defines three key steps in the periodic self-organization of neuromasts in the primordium: first, a step that polarizes Wnt activity in the primordium; second, a pattern-forming step that generates periodic Fgf–signaling centers in the context of polarized Wnt activity; and third, a step involving Sox2, which helps stabilize fate and morphology in neuromasts formed in the earlier pattern forming stage.
Although the steps outlined above are specific to the way the primordium develops, they define more broadly the sequence of events in the self-organization of development in many different contexts. A symmetry-breaking event that polarizes a tissue is a common first step in most developmental processes, which then provides the context for self-organizing mechanisms that determine patterning of cell fate, morphology, and cell migration within the tissue. Although self-organizing mechanisms could generate pattern spontaneously, independently of tissue polarization, their operation in the context of prior polarization ensures a more predictable reproducible outcome. Furthermore, while a chemical signaling–based reaction diffusion system has been described above as an example of a pattern-forming system, pattern formation can operate at many scales with different modalities. For example, our previous studies showed that the number and size of rosettes in the primordium can also be understood as an outcome of mechanical interactions between cells with periodic aggregates forming as a consequence of a balance of forces, such as apical constriction that pull cells together to form rosettes, while tension along the length of the primordium, associated with collective cell migration, pulls cells apart, opposing formation of rosettes. These modalities operate in parallel, interact, and typically work synergistically to determine robust reproducible outcomes. Patterns initiated by such self-organizing mechanisms are not necessarily stable and, as seen in the example of the lateral line primordium, are followed by changes in regulation that help consolidate and stabilize form and fate established in an earlier patterning phase.
Wnt–dependent BMP expression inhibits rosette formation at the leading end of the primordium.
As stated above, the zebrafish pLLp migrates from the ear to the tip of the tail, periodically forming and depositing neuromasts. Protoneuromasts form as clusters of primordium cells, epithelialize, apically constrict, and form rosettes. Formation of epithelial rosettes is promoted by periodic formation of Fgf–signaling centers, starting from the trailing end of the primordium. Fgf signaling promotes rosette formation by promoting apical basal polarization of cells and by determining expression of factors, such as the protein Shroom3, that promote apical constriction. Conversely, Wnt signaling, which dominates in the leading part of the primordium, inhibits rosette formation, in part by promoting expression of factors such as Dusp6 and Sef1, which prevent Wnt–active cells from responding effectively to Fgf. However, the observation that the growth factors Bmp 2b, Bmp 4, and Bmp 5 are expressed in the leading part of the primordium under the influence of Wnt signaling suggested that Bmp signaling, previously known to promote EMT (epithelial-mesenchymal transition), may contribute to maintenance of the quasi-mesenchymal morphology of leading cells and to inhibition of rosette formation.
Consistent with a role for Bmps in inhibiting the formation of rosettes, exposing the embryos to a BMP inhibitor, K02288, allowed cells in the leading zone to aberrantly form epithelial rosettes. However, although K02288 was characterized as a potent inhibitor of the type I Bmp receptor Alk2, aberrant rosette formation in leading cells was not observed in an Alk2 mutant, or following knockdown of Bmp5, the Bmp expressed in the primordium that was thought to signal via the Alk2. We reported that exposure to DMH2, another Bmp inhibitor with greater specificity for Alk3 and Alk6, reproduces the aberrant rosette formation originally seen with K02288, which has a limited ability to inhibit these Type I receptors as well. This observation suggested that inhibition of rosette formation in the leading zone was not determined by Bmp5 but instead by Bmp2b and Bmp4, also expressed in the primordium and known to preferentially signal via Alk3 and Alk6. Consistent with this interpretation, knockdown of Alk6 also allowed rosettes to form aberrantly in the leading zone. Conversely, induced ectopic expression of Bmp2b inhibited rosette formation, resulting in a broader leading zone without rosettes in the migrating primordium.
Inhibition of Bmp signaling with K02288 has no obvious effect on the pattern of Wnt and Fgf signaling in the primordium, suggesting that Bmp normally operates downstream of these signaling pathways to inhibit rosette formation in the leading zone. Despite the absence of any change in Fgf signaling, we found that inhibiting Bmp signaling with K02288 allows shroom3, previously thought to be regulated by Fgf signaling, to be aberrantly expressed closer to the leading end of the primordium. Expansion of shroom3 expression into the leading zone was accompanied by loss of DUSP6 expression in this domain. DUSP6 is a dual activity phosphatase that inhibits phospho–ERK function downstream of Fgf signaling. However, previous studies had suggested that DUSP6 expression is regulated by Lef1 downstream of Wnt signaling, Together, these observations suggested that Lef1 operates downstream of Wnt signaling to determine Bmp expression, which in turn promotes DUSP6 expression to prevent rosette formation in the leading Wnt–active zone. Consistent, with this hypothesis, we showed that lef1 regulates Bmp expression. Furthermore, knockdown of lef1 results in premature formation of rosettes closer to the leading end, recapitulating effects of inhibiting Bmp signaling.
Collaborators
- Harshad Vishwasrao, PhD, Advanced Imaging and Microscopy Resource, NIBIB, Bethesda, MD
Contact
For more information, email chitnisa@mail.nih.gov or visit https://www.nichd.nih.gov/research/atNICHD/Investigators/chitnis.