Chromatin Remodeling and Gene Activation
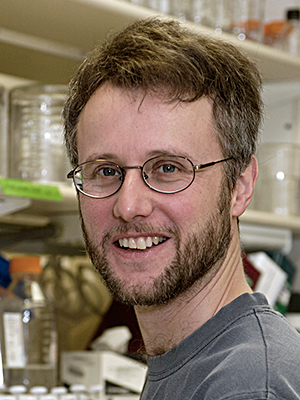
- David J. Clark, PhD, Head, Section on Chromatin and Gene Expression
- Peter Eriksson, PhD, Staff Scientist
- Christopher Coey, PhD, Intramural Research Training Award Fellow
- Hemant K. Prajapati, PhD, Visiting Fellow
- Zhuwei Xu, PhD, Visiting Fellow
Aberrant gene regulation is the basis of many disease states. Our main objective is to understand how genes are activated for transcription in the context of chromatin structure. Chromatin is not just a packaging system for DNA in eukaryotic cells; it also participates in gene regulation. The structural subunit of chromatin is the nucleosome, which contains nearly two turns of DNA coiled around a central-core histone octamer. Nucleosomes are generally quite regularly spaced along the DNA, like beads on a string. Gene regulation involves either attenuation of the inherently repressive properties of nucleosomes to facilitate gene expression, or enhancement of those properties to ensure complete repression. These events are choreographed by DNA sequence–specific transcription factors (activators and repressors) and chromatin remodeling complexes. The latter can be divided into two groups: histone- or DNA–modifying enzymes that implement the “epigenetic code”, and ATP–dependent remodeling machines, which move or displace nucleosomes. We are exploiting and developing high-throughput technologies to obtain genome-wide maps of nucleosomes, chromatin-remodeling complexes, and RNA polymerase II in budding yeast to determine how chromatin organization is affected when genes are activated. The current objectives of our yeast studies are: (1) to determine the roles of the various chromatin-remodeling complexes (RSC, SWI/SNF, ISW1, ISW2, CHD1, INO80C) in chromatin organization and gene expression, why there are so many different remodelers, and whether they are functionally redundant; our studies so far indicate that each remodeling enzyme makes a different contribution to chromatin organization and is important because genes encoding subunits of some of these enzymes are often mutated in various cancers; (2) to test the hypothesis that nucleosomes control DNA accessibility and play a vital role in gene regulation by blocking promoters. We have developed a new method (qDA-seq) to obtain quantitative measurements of genome accessibility in nuclei and in living cells, using budding yeast and human or mouse cell lines as model systems.
Measuring genome accessibility in living cells
Gene activation involves the recruitment of a set of factors to a promoter in response to appropriate signals, ultimately resulting in the formation of an initiation complex by RNA polymerase II and transcription. These events coincide with the removal of promoter nucleosomes to create a nucleosome-depleted region (NDR). This observation has led to the generally accepted model that promoter nucleosomes physically block transcript initiation, acting as repressors by preventing access to specific transcription factor–binding sites. The nucleosome is a highly stable structure containing tightly wound DNA, which is largely inaccessible to sequence-specific DNA–binding proteins. Activation occurs if sequence-specific ‘pioneer’ transcription factors (proteins that bind to nucleosomal sites with high affinity) are present, and/or if ‘classical’ transcription factors, which are normally blocked by nucleosomes, recruit ATP–dependent chromatin remodelers to move or evict promoter nucleosomes, thus facilitating initiation complex formation.
The ATP–dependent chromatin remodelers either move nucleosomes along DNA, remove the histones altogether, or form arrays of regularly spaced nucleosomes. Examples include the SWI/SNF and RSC chromatin structure remodeling complexes, which remodel nucleosomes on genes and at promoters, and the CHD and ISWI remodeling complexes, some of which are involved in determining nucleosome spacing. The INO80C remodeling complex is unusual because it appears to have both properties. Human diseases have been linked to chromatin remodeling enzymes. For example, mutations in the hSNF5 subunit of the SWI/SNF complex are strongly linked to pediatric rhabdoid tumors, and the CHD remodelers have been linked to cancer and autism. Therapies and drugs aimed at epigenetic targets are being tested. Thus, a full understanding of chromatin structure and the mechanisms by which it is manipulated is vital.
The general view is that nucleosomes and chromatin structure play a central role in gene regulation by restricting access to genomic DNA (Figure 1). Experiments measuring DNA accessibility in nuclei have established that nucleosomes block access to DNA (e.g., by MNase-seq, ATAC-seq, or qDA-seq). Studies from many groups have confirmed this observation and is stated as a fact in the introductions to most chromatin papers (including our own) and in reviews of the chromatin field. Indeed, the observation that nucleosomes block access to DNA is central to current models of gene regulation, as mentioned above. However, nuclei might not represent an accurate model for living cells, because chromatin dynamics may be “frozen” when cells are disrupted, which results in the loss of metabolites, such as the ATP required for remodeling.
Figure 1. DNA packaging in the nucleus: to what extent does chromatin compaction limit access to the DNA?
DNA is packaged into the nucleus by histones. The basic structural subunit of chromatin is the nucleosome core, which contains about 147 bp of DNA wrapped nearly twice around a central octamer of core histones. Nucleosomes are regularly spaced along the DNA like beads on a string; the intervening DNA is called the linker DNA and is bound by linker histone (H1). The beads-on-a-string fiber spontaneously condenses into a heterogeneous fiber of about 30 nm width. Genomic regions rich in repetitive elements form constitutive heterochromatin in all cells, in which the chromatin fiber is even more condensed. Facultative heterochromatin is formed on genes that should be permanently silent in a specific differentiated cell type. Heterochromatin is densely packed and darkly staining in the electron micrograph shown here. Euchromatin is less condensed (light staining) and contains active genes. We are interested in determining to what extent chromatin limits DNA accessibility. Figure adapted from Chereji et al. Genome Res 2019;29:1985-1995.
To address this question, we are comparing the accessibility of DNA genome-wide in nuclei and in living yeast cells using DNA methylases. Remarkably, we find that the genome is globally accessible in living cells, unlike in nuclei. That is, nucleosomes do not block access to genomic DNA in vivo. We find that methylation by the Dam DNA methylase (which methylates A in the sequence GATC) is blocked by nucleosomes in isolated nuclei. However, expression of Dam using an inducible promoter results in methylation of the entire genome in living cells, with minimal interference from nucleosomes. Using a different DNA methylase, M.SssI (which methylates C in CG), together with Nanopore long-read sequencing, we showed that centromeric nucleosomes, unlike canonical nucleosomes, are exceptionally stable, protecting their DNA from methylation in vivo. We also observe that at least three ATP–dependent chromatin remodelers (RSC, ISW1, and CHD1) contribute to nucleosome dynamics in vivo, using a degron approach to detect nucleosome movements in living cells as remodelers are depleted.
Our data demonstrate that nucleosomes are in a continuous state of flux in living cells, but static in nuclei, presumably resulting from loss of critical factors during isolation. This flux may involve nucleosome sliding, nucleosome removal and replacement, and/or nucleosome conformational changes, catalyzed by ATP–dependent chromatin remodelers (Figure 2). We propose that the various remodelers compete with one another in vivo, continually moving nucleosomes to different positions, resulting in a nucleosome flux that renders the yeast genome essentially transparent to transcription factors and other DNA–binding proteins. Our observations have profound implications for the chromatin field, requiring a re-examination of the roles of the chromatin remodelers in gene regulation, and of the extent to which packaging the genome into nucleosomes is actually repressive. A revised manuscript describing our data has been submitted. Currently, we are extending our studies to human cell lines.
Figure 2. Chromatin flux model to explain the difference in genome accessibility in nuclei and living cells
We propose that nucleosomes are stationary in nuclei, protecting the DNA they contain (top panel). The Dam DNA methyltransferase only methylates the short stretches of linker DNA between regularly spaced nucleosomes. However, in living cells, Dam methylates virtually the entire genome (bottom panel), because the nucleosomes are in flux. Nucleosomes may be continuously removed and replaced, or shunted from side to side, or opened up, as shown, resulting in DNA exposure and methylation. We propose that the flux is generated by the competing activities of the various ATP–dependent chromatin remodelers (such as RSC, SWI/SNF, ISW1, and CHD1).
Long-read sequencing methods, namely the PacBio and Nanopore platforms, are becoming increasingly popular, as the power of these new technologies is fully appreciated. In our own field of chromatin biology, epigenetic modifications such as m5C and m6A can now be detected directly in original (i.e., unamplified) multi-kilobase DNA molecules. A recent extension of this approach is the use of methylation footprinting, in which an exogenous DNA methylase is added to nuclei or chromatin, where it methylates accessible regions, such as nucleosome-depleted promoters and the linkers between nucleosomes, but not nucleosomal DNA, which it cannot access. The result is a methylation map of each molecule, to be interpreted in terms of footprints and accessible regions.
We performed methylation footprinting in budding yeast nuclei using M.EcoGII, an adenine-specific DNA methylase (Figure 3). The potential footprint resolution of this approach is very high because of the adenine density of DNA. We used PacBio long-read sequencing to detect m6A introduced by M.EcoGII, and we developed a pipeline to analyze the data. We discovered a number of critical, previously unreported, issues concerning PacBio methylation footprinting data, which must be corrected before an accurate chromatin map can be obtained. Specifically, we observed low-limit methylation levels in the genomic DNA–positive controls, a wide range in the fraction of m6A from one DNA molecule to the next, and a strong local bias against methylation of AT–rich sequences and poly(A) runs. We also found that there is a high probability of observing a single m6A base within a nucleosome, breaking up the expected around 147 bp footprint. Our novel probability model resolves all of these critical interpretative problems. The pipeline output includes an IGV–ready bam file, which displays both called m6A bases, and our interpretation of the methylation pattern as accessible regions and nucleosomes in individual DNA molecules.
Figure 3. Mapping nucleosomes on single DNA molecules using methylation footprinting and PacBio long-read sequencing
The M.EcoGII DNA methyltransferase methylates adenine bases in DNA, converting them into 6-methyladenine (m6A).
(A) Nuclei are incubated with M.EcoGII, which methylates adenines in accessible DNA, including nucleosome-depleted regions at gene promoters and the short linkers between nucleosomes. Nucleosomal DNA is protected from methylation by the histones.
(B) After purification, genomic DNA is fragmented into molecules of several thousand base pairs, and sequenced using PacBio technology; m6A can be detected because the PacBio–sequencing polymerase pauses when it encounters m6A in the template strand. The result is a methylation map corresponding to accessible regions, which can be interpreted in terms of nucleosome footprints. Each mapped DNA molecule represents a stretch of DNA from a single cell. By comparing multiple molecules containing the same sequence, we detected extensive cell-to-cell variation in nucleosome positioning.
We used our data to investigate heterogeneity in chromatin structure around the transcription start sites of yeast genes. We found that nucleosome positioning on a specific gene varies widely from cell to cell, with only a small fraction of genes showing a similar ordered nucleosomal array in every cell, even though the cell population average is as expected from other techniques, such as MNase-seq. We quantify the degree of heterogeneity for every yeast gene using a novel correlation score. A revised manuscript describing our data has been submitted.
Collaborators
- Jeffrey J. Hayes, PhD, University of Rochester Medical Center, Rochester, NY
- Alan Hinnebusch, PhD, Section on Nutrient Control of Gene Expression, NICHD, Bethesda, MD
- David Levens, MD, PhD, Laboratory of Pathology, Center for Cancer Research, NCI, Bethesda, MD
- Karel Pacak, MD, PhD, DSc, Section on Medical Neuroendocrinology, NICHD, Bethesda, MD
- Vasily M. Studitsky, PhD, Fox Chase Cancer Center, Philadelphia, PA
Contact
For more information, email clarkda@mail.nih.gov or visit https://www.nichd.nih.gov/research/atNICHD/Investigators/clark.