Hippocampal Interneurons and Their Role in the Control of Network Excitability
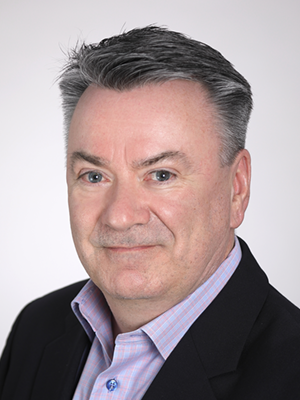
- Chris J. McBain, PhD, Head, Section on Cellular and Synaptic Physiology
- Ramesh Chittajulla, PhD, Staff Scientist
- Edra London, PhD, Staff Scientist
- Kenneth Pelkey, PhD, Staff Scientist
- Adam Caccavano, PhD, Postdoctoral Fellow
- June Hoan Kim, PhD, Postdoctoral Fellow
- Geoffrey Vargish, PhD, Postdoctoral Fellow
- Steven Hunt, BS, Biologist
- Xiaoqing Yuan, MSc, Biologist
- Erica Lesko, BS, Postbaccalaureate Fellow
- Anna Vlachos, BSc, Postbaccalaureate Fellow
Cortical and hippocampal GABAergic inhibitory interneurons (INs) are ‘tailor-made’ to control cellular and network excitability by providing synaptic and extrasynaptic input to their downstream targets via GABAA and GABAB receptors. The axons of this diverse cell population make local, short-range projections (although some subpopulations project their axons over considerable distances) and release the inhibitory neurotransmitter gamma-aminobutyric acid (GABA) onto a variety of targets. Distinct cohorts of INs regulate sub- and supra-threshold intrinsic conductances, regulate Na+- and Ca2+-dependent action-potential generation, modulate synaptic transmission and plasticity, and pace both local and long-range, large-scale synchronous oscillatory activity. An increasing appreciation of the roles played by INs in several neural-circuit disorders, such as epilepsy, stroke, Alzheimer’s disease, and schizophrenia, has seen this important cell type take center stage in cortical circuit research. With almost 30 years of interest in this cell type, the main objectives of the lab have been to understand: (1) the developmental trajectories taken by specific cohorts of INs as they populate the nascent hippocampus and cortex; (2) how ionic and synaptic mechanisms regulate the activity of both local-circuit GABAergic INs and principal neurons (PN) at the level of small, well defined networks; and (3) how perturbations in their function alter the cortical network in several neural-circuit disorders. To this end, we use a variety of electrophysiological, imaging, optogenetic, immunohistochemical, biochemical, molecular, and genetic approaches with both wild-type and transgenic animals.
Figure 1. Hippocampal GABAergic terminals
Three major types of GABAergic terminal are shown in the hippocampal dentate gyrus (red, blue, and green punch). The black negative space shows the cell bodies of principal cells innervated by the GABAergic terminals.
Background and significance
In hippocampus, GABAergic local circuit inhibitory INs account for about 10–15% of the total neuronal cell population. Despite being in the minority, this diverse neuronal population serves as a major determinant of all aspects of cortical circuit function and regulation. Within the hippocampus, INs have their cell bodies scattered across all major subfields, and the positioning of their somatodendritic arbors allows integration of input from a number of intrinsic and extrinsic afferent inputs. The axons of many IN subtypes largely remain local to the subfield housing their soma and dendrites; however, many form long-range projections that extend beyond their original location to ramify within both cortical and subcortical structures. Their axons target well defined narrow postsynaptic domains (i.e., soma and proximal dendrites) or can provide widespread input to large portions of target cell dendrites. This innervation of different postsynaptic cellular compartments ensures that virtually all domains of their principal neuron targets receive extensive coverage and, importantly, underscores that IN subtypes perform distinct roles in the hippocampal circuit. INs are primarily providers of inhibitory GABAergic synaptic input: a physiological role that utilizes Cl– influx or K+ efflux via cognate GABAA or GABAB receptor activation respectively, to transiently hyperpolarize or shunt the cell membrane away from action potential threshold. They play major roles in not only the regulation of single cell excitability, but provide well timed inhibitory input that dictates the temporal window for synaptic excitation, and subsequent action potential initiation, thus shaping the timing of afferent and efferent information flow. In addition, they harness and synchronize both local and distributed cortical circuits to facilitate oscillatory activity across broad frequency domains. Indeed, several developmentally regulated neural circuit disorders, such as epilepsy, schizophrenia, and autism, are likely associated with deficits in the numbers and function of distinct IN cohorts. For all of these reasons, INs have recently become the intense focus of investigators drawn from a wide variety of backgrounds.
In the past year, our research focused on three main aspects of IN function. (1) We continued our study of glutamatergic and GABAergic synaptic transmission made onto and from INs and their downstream targets, within the hippocampal and cortical formations. (2) We capitalized and expanded our research using genetic and viral approaches to examine the development of specific cohorts of medial- and caudal-ganglionic eminence–derived INs and their roles in both nascent and mature circuits. (3) As part of a multi-institute non-human primate consortium, we expanded our studies to consider evolutionary conservation or diversity of principal neuron and interneuron function, using tissue derived from rodent, non-human primates (macaque and marmoset) and, in collaboration with NINDS neurosurgery, surgically resected human hippocampal and cortical tissue. This multiparametric research approach to circuit development and function has been extremely fruitful and is a perfect example of why our research strategy is well suited for the intramural environment. Having the flexibility to pursue this line of research would not have been possible without the support and collaborative nature of the NIH intramural program.
Figure 2. MGE– and CGE–derived hippocampus interneurons
The figure shows the major cortical and hippocampal inhibitory interneuron subtypes derived from both the medial and caudal ganglionic eminences.
Evolutionary conservation of hippocampal mossy fiber synapses
Computational models based primarily on rodent data predict that mossy fiber (MF) synaptic connections between hippocampal dentate gyrus granule cells (DGGCs) and CA3 pyramidal cells (PCs) are essential for encoding contextual memories. MF encoding of memory is considered to relate directly to a host of peculiar specialized structural/functional synaptic properties, including sparse innervation by large multi-release site terminals supporting a remarkable frequency-dependent dynamic range of transmission onto the most proximal dendrites of CA3 PCs. Though investigated in exquisite detail in model organisms, synapses, including MFs, have undergone relatively minimal functional interrogation in the human brain. To determine the translational relevance of rodent MF findings to the human brain, we evaluated the basic synaptic properties of MF connections within human hippocampal tissue resected for treatment of pharmaco-resistant epilepsy. Human MF transmission exhibits remarkably similar hallmark features to that of rodents, including AMPA–receptor dominated synapses with small contributions from NMDA and kainate receptors, large dynamic range with strong frequency facilitation, NMDA receptor–independent presynaptically expressed long-term potentiation, and strong cAMP sensitivity of presynaptic release. Moreover, serial array tomography electron microscopy confirmed evolutionary conservation of MF synapse ultrastructure. The astonishing congruence of the core features shared between rodent and human MF synapses argues that the basic properties of MF transmission reported in experimental animal models are also critical to human MF function. However, of interest from the disease perspective, we observed a dramatic selective deficit in GABAergic inhibitory tone onto human MF postsynaptic targets, suggesting that unrestrained detonator excitatory drive contributes to circuit hyperexcitability in epilepsy.
Development of tools for evolutionary conservation
Over the last few years, we have begun to explore evolutionary conservation of both principal neuron and interneuron function from rodents through non-human primates to humans. This has necessitated the generation of tools that allow unequivocal identification of INs in species from which few studies exist. In two ongoing collaborations with the labs of Gordon Fishell and Matt Rowan, we developed and characterized several AAV (adeno-associated virus) vectors utilizing neuron type–specific regulatory transcriptional sequences (enhancer-AAVs), which have allowed us to overcome the limitations set by using mouse Cre-lines. The ability to precisely control transgene expression is essential for basic research and clinical applications. AAVs are non-pathogenic and can be used to drive stable expression in virtually any tissue, cell type, or species, but their limited genomic payload results in a trade-off between the transgenes that can be incorporated and the complexity of the regulatory elements controlling their expression. Resolving these competing imperatives in complex experiments inevitably results in compromises. We achieved this in compact vectors by integrating structural improvements of AAV vectors with innovative molecular tools. In a series of manuscripts, we illustrated the potential of this approach through a systematic demonstration of their utility for targeting cell types and querying their biology, using a wide array of genetically encoded tools. These enhancer viral tools were used in many of the studies described above and have proved of great utility in mouse, marmoset, macaque, and human tissue. Our hope is to continue this fruitful avenue of collaboration for future experimental use.
Additional Funding
- Connie Mackenzie Gray-Scott was funded by an NIH-Wellcome Trust Graduate Student award.
Publications
- Pelkey KA, Vargish G, Pellegrini L, Calvigioni D, Chapeton J, Yuan X, Hunt S, Cummins AC, Eldridge MAG, Pickel J, Chittajallu R, Averbeck BB, Toth K, Zaghloul K, McBain CJ. Evolutionary conservation of hippocampal mossy fiber synapse properties. Neuron 2023 111(23):3802–3818.e5.
- Mahadevan V, Mitra A, Zhang Y, Yuan X, Peltekian A, Chittajallu R, Esnault C, Maric D, Rhodes C, Pelkey KA, Dale R, Petros T, McBain CJ. NMDARs drive the expression of neuropsychiatric disorder risk genes within GABAergic interneuron subtypes in the juvenile brain. Front Mol Neurosci 2021 14:712609.
- Xiao MF, Roh SE, Zhou J, Lucey B, Craig MT, Hays L, Jia M, Chien CC, Xu D, Zhou W, Talbot C, Weinberg D, Sawa A, Pelkey KA, McBain CJ, Savonenko A, Worley PF. A biomarker-authenticated model of schizophrenia implicating NPTX2 loss of function. Sci Adv 2021 7(48):eabf6935.
- Mackenzie-Gray Scott CA, Pelkey KA, Caccavano A, Abebe D, Lai M, Black KN, Brown N, Trevelyan AJ, McBain CJ. Resilient hippocampal gamma rhythmogenesis and parvalbumin-expressing interneuron function before and after plaque burden in 5xFAD Alzheimer’s disease model. Front Synaptic Neurosci 2022 14:857608.
- Pouchelon G, Vergara J, McMahon J, Gorissen B. Lin J, Vormstein-Schneider D, Niehaus JL, Burbridge T, Wester JC, Sherer M, Fernandez-Otero M, Allaway K, Pelkey K, Chittajallu R, McBain CJ, Fan M, Nasse J, Wildenberg J, Fishell G, Dimidschstein J. A versatile viral toolkit for functional discovery in the nervous system. Cell Rep Methods 2022 2:100225.
Collaborators
- Jordan Dimidschstein, PhD, The Broad Institute, Cambridge, MA
- Gordon Fishell, PhD, The Broad Institute, Cambridge, MA
- Timothy J. Petros, PhD, Unit on Cellular and Molecular Neurodevelopment, NICHD, Bethesda, MD
- Mathew Rowan, PhD, Emory University, Atlanta, GA
- Kareem Zaghloul, MD, PhD, Functional and Restorative Neurosurgery Unit, NINDS, Bethesda, MD
Contact
For more information, email mcbainc@mail.nih.gov or visit https://www.nichd.nih.gov/research/atNICHD/Investigators/mcbain.