Mechanisms of Synapse Assembly and Homeostasis
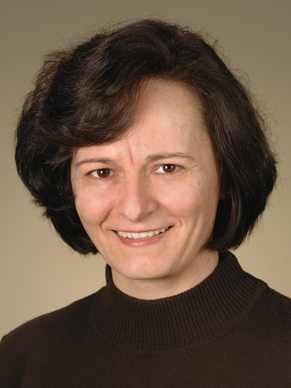
- Mihaela Serpe, PhD, Head, Section on Cellular Communication
- Peter Nguyen, Biological Laboratory Technician
- Tae Hee Han, PhD, Staff Scientist
- Rosario Vicidomini, PhD, Research Fellow
- Wen Chieh Hsieh, PhD, Visiting Fellow
- Tho Huu Nguyen, PhD, Visiting Fellow
- Debabrata Sinha, PhD, Visiting Predoctoral
- SiJia Chen, BSc, Postbaccalaureate Fellow
- Thomas Brody, PhD, Special Volunteer
The purpose of our research is to understand the mechanisms of synapse development and homeostasis. The chemical synapse is the fundamental nervous-system communication unit that connects neurons to one another and to non-neuronal cells and is designed to mediate rapid and efficient transmission of signals across the synaptic cleft. Crucial to this function is the ability of a synapse to change its properties, so that it can optimize its activity and adapt to the status of the cells engaged in communication and/or to the larger network comprising them. Consequently, synapse development is a highly orchestrated process coordinated by intercellular communication between the pre- and postsynaptic compartments and by neuronal activity itself. Our long-term goal is to elucidate the molecular mechanisms that regulate assembly of functional synapses during development and their fine-tuning during maturation, plasticity, and homeostasis. We use a comprehensive set of approaches that include genetics, biochemistry, molecular biology, cell biology super-resolution imaging, and electrophysiology recordings in live animals and in reconstituted systems. In recent studies, we also utilized single-cell RNA sequencing (scRNA-seq) methodologies to describe various populations of neurons.
Because of its many advantages, we use the Drosophila neuromuscular junction (NMJ) as a model for glutamatergic synapse development and function. The fact that individual NMJs can be reproducibly identified from animal to animal and are easily accessible for electrophysiological and optical analysis makes them uniquely suited for in vivo studies on synapse assembly, growth, and plasticity. In addition, the richness of genetic manipulations that can be performed in Drosophila permits independent control of individual synaptic components in distinct cellular compartments. Importantly, the fly NMJ relies entirely on kainate-type receptors, a family of ionotropic glutamate receptors that impact synaptic transmission and neuronal excitability in the mammalian central nervous system but remain poorly understood. The Drosophila NMJ can thus be used to analyze and model defects in the structural and physiological plasticity of glutamatergic synapses, which are associated with a variety of human pathologies, from learning and memory deficits to autism. Drosophila has long served as a source of insight into human genetics, development, and disease. The basic discoveries of our laboratory makes in the fly will serve our overarching goal of understanding how chemical synapses are assembled and sculpted during development and homeostasis.
Cellular diversity in the Drosophila third instar larval ventral cord revealed by single-cell transcriptomics
In flies, as in vertebrates, neuronal activity induces input-specific changes in the synaptic strength; at the larval NMJ, the postsynaptic sensitivity is primarily modulated via synapse-specific recruitment of postsynaptic glutamate receptors. Robust homeostatic mechanisms keep synapses within an appropriate dynamic range such that the evoked potentials measured in the muscle remain constant from embryo to third instar larvae.
The nervous system has a bewildering array of cells with massive diversity. The diversity of cell identity translates into functional diversity, with different types of cells working in concert to perform different tasks. We wish to understand how this diversity develops and how is it harnessed for function. A highly choreographed division of labor ensures that the nervous system functions properly. However, the number of different cell types involved and how we define these cells and their contributions are still open questions.
The fruit fly nervous system is a powerful model system for finding order in this complexity. Much has been learned by studying subsets of neurons and/or circuits one by one. Defining neuronal diversity feature by feature has been informative but has yielded limited information. A wealth of specific information has been collected, but the general themes are hard to discern, and it is impossible to tell what is missing. Single-cell RNA-seq approaches promise to fill this gap. The Drosophila larval ventral nerve cord (VNC) is the ideal system in which to conduct such work, because it is complex enough to sustain coordinated locomotor behaviors but also small enough to enable thorough analyses. Following a first wave of neurogenesis, the fly embryos hatch into the first instar larval stage with a central nervous system (CNS) consisting of two brain lobes and a VNC, with an estimated 10,000 cells. The reactivation of neuroblasts during the larval stage allows the VNC to expand to over 100k cells as the larva progresses to adult stages. The crawling larva and the flying adult have different food preferences, different predators, and use dramatically different sensory modalities and locomotor circuits to perform their specific behaviors. Our questions are what the relationship is, if any, between the nervous system of the larva and adult and how many of the cells born during larval stages are added to augment larval function and how many are made to serve dedicated, non-larval functions in the adult animal. Do larval and adult neurons have common features and if yes, how do they relate to their specific functions? Is the nature of glia diversity similar or different from neurons? Which features are idiosyncratic to a given cell and which ones are indicative of general principles of circuit development and function? In the past years we addressed some of these questions by scRNA-seq analyses of the larval VNC. During this process, we adapted and developed new protocols for dissociating single cells from fly larvae, then assembled a custom multistage analysis pipeline that integrates modules contained in different R packages to ensure flexible, high-quality RNA-seq data analysis. This work was conducted with support from the NICHD Genomics Core and in collaboration with Steve Coon, Fabio Faucz, and James Iben.
In brief, we dissected third instar larvae VNCs, dissociated the cells, and sequenced over 31,000 high-quality single cells. Using un-supervised clustering algorithms, we clustered the cells into distinct populations. We then assigned the populations to specific cell types using known markers. We found that only 40% of the larval VNC cells (13,120) are mature and could fulfill larval functions. The other 60% cells (17,920) are dedicated to setting up the VNC cell populations needed later, during adult stages. These immature cells include neural progenitors called neuroblasts (NBs), and their progenies that will become the future adult neurons. Using defining genetic markers, we captured a developmental trajectory that included neuroblasts (marked by the presence of dpn, mira, wor, and ase), their immediate progeny ganglion mother cells (GMCs) (wor, ase), newborn neurons (Hey), and immature secondary neurons at two distinct developmental stages, first with lower levels of Imp (jim, dati, pros, and low Imp) and second with high levels of Imp expression (mamo and high Imp) (Figure 1).
Figure 1. Major cell types in third instar larval VNC
A. Two-dimensional representation (UMAP; uniform manifold approximation and projection) of 31,040 cells from five different control sets. Each dot represents a single cell. Each cell type was assigned based on expression of known markers and illustrated by unique color.
B. Dot plot of marker genes for each major cell type. The dot size represents the percent of cells that express each gene of interest (GOI) within the cluster. The color intensity reflects average expression level.
Two clusters, identified by specific motor neuron (MN) markers (futsch, VGlut, lbm, Proc, rhea), illustrate the essence of the larval VNCs divide: one population (primary larval MNs) is metabolically active and expresses all the components of active, functional synapses while a second population (secondary MNs) is metabolically and functionally silent. Upon finding their target muscles, primary MNs receive retrograde, muscle-derived BMP (bone morphogenetic protein) signals, which modulate transcription and coordinate synapse development. Indeed, primary MNs express BMP target genes such as twit (target of wit); in contrast, immature secondary MNs show no twit expression, suggesting they lack retrograde BMP signaling at this stage. A large cluster of putative interneurons contains heterogenous neurons that are metabolically and functionally active during third instar larvae and express distinct neurotransmitter markers (VGlut, ChAT, Gad1, and DAT). We also identified many glia subtypes, including a glial precursor (marked by gcm), midline glia (sli, comm, wrapper), subperineurial glia (Mdr65), cortex glia (wrapper, zyd), astrocytes (alrm), and ensheathing glia (Eaat2). Besides glia and neurons, we also identified a cluster of trachea cells, characterized by the expression of verm.
These initial assessments indicate the richness of our atlas and its capacity to distinguish among different cell types, cell states, and functional characteristics within the larval VNC.
A developmental trajectory of secondary neurons
The high proportion of immature secondary neurons (60% of the total) in the third instar larval VNC and their apparent organization into a developmental trajectory prompted us to examine whether some of these neurons are already specified and have acquired their cell identities. We re-clustered these cells, applied different pseudotime algorithms, including slingshot, diffusion map, and monocle 2, and found that all algorithms placed these cell clusters into a pseudotime trajectory with excellent correlation scores (Figure 2). We found that peak expression for five previously described cell identifiers (dpn, ase, Hey, pros, Imp), which follow the progression from type I NBs to secondary neurons, aligned perfectly within this developmental trajectory. In our dataset, each of the cell populations along the trajectory are extremely well defined; this provides new insights in the respective cell functions.
By third instar larval stage, secondary neurons have already acquired cell-fate determinants and have committed to distinct neurotransmitter identities (Figure 2). We found that immature neuron clusters can be grouped unambiguously based on (1) distinct neurotransmitter markers such as vGlut (vesicular glutamate transporter), Chat (choline acetyltransferase) or Gad1 (glutamic acid decarboxylase, an enzyme involved in GABA synthesis) and (2) different combinations of transcription factors. For example, glutamatergic neurons in clusters 5 and 2 are enriched in Ets65A, HLH3B, aop, and E5; GABAergic neurons in clusters 3, 4, and 9 express HLH4C, sp1, exex, salr, and scro; finally, cholinergic neurons in clusters 8 and 4 are enriched in slou, unc-4, acj6, and kn. These observations indicate that the secondary neurons within the developmental trajectory are already committed to different cell identities.
Figure 2. A developmental progression from neuroblasts to immature secondary neurons
A. UMAP plot of developmental trajectory clusters reveals five major developmental states.
B. Trajectory clusters ordered along a pseudotime calculated by Slingshot;
C. Classic diagram of developmental progression from type I neuroblasts to newborn neurons;
D. Pseudo-temporal expression of established markers for the five major stages;
E–G. Dot and density plots illustrating that the larval secondary neurons are already committed to different cell fates, including neurotransmitter identities.
Larval and adult postembryonic neurons share common features
To examine what proportion of these cell identities is maintained in the adult stages and whether the development of the adult VNC involves profound changes that alter the identities of these already committed cells, we compared the transcriptional profiles of secondary neurons from larval VNC with the adult VNC neurons, as derived from the published dataset. First, we used unsupervised clustering algorithms to classify the cells in adult VNC and manually annotated these cells into known hemilineages based on their cell identities (Figure 3). To capture more diversity within the larval secondary neurons, we sub-clustered these populations using more principal components (PCs) (45 total). Using the adult hemilineages as a reference dataset, we then combined two machine-learning algorithms (support vector machine and random forest) included in the ELeFHAnt R package, to uncover any similarity between postembryonic neurons in larval and adult VNCs. Remarkably, these algorithms predicted that transcriptional profiles of 20 out of 21 adult hemilineages have high similarities with discrete subclusters of secondary larval neurons (predicted larval hemilineages). The remaining hemilineage, lin15B, was defined as adult MNs and corresponds to a well defined larval cluster outside the trajectory cells, called here secondary MNs.
Figure 3. Most adult neurons acquire cell-fate identity during larval stages.
A. tSNE plot showing reference hemilineages from the published adult VNC RNA-seq dataset; each hemilineage was annotated based on already known markers.
B. tSNE plot of re-clustered larval secondary neurons (without NBs, GMCs, or Hey populations);
C. tSNE plot indicating predicted larval VNC hemilineages, using the adult dataset as reference;
D. Correlogram comparing adult reference hemilineages and larval secondary neuron sub-clusters (as shown in B).
To confirm the identity between predicted larval hemilineages and reference adult hemilineages, we examined their differential gene expression. We found that expression of marker genes in each hemilineage is strikingly similar between adult and larval stages, starting with combinatorial arrays of transcription factors, which are the cell-fate determinants for individual hemilineages. The larval and adult clusters are also perfectly matched in their neurotransmitter identities and even in the signaling networks they engage. We searched for additional hemilineages within the adult VNC dataset and uncovered at least three new lineages with highly similar gene expression. Furthermore, when cells within each hemilineage were highlighted within UMAP (uniform manifold approximation and projection) plots, we noted that, with maybe one exception (lin13B), all hemilineages follow the developmental trajectory described above and include newborn and progressively differentiating neurons. Such distributions are consistent with larval hemilineages being actively engaged in a second wave of neurogenesis.
In contrast to adult lineages, larval secondary neurons have low levels of glycolysis-related transcripts, indicative of metabolically low or inactive neurons. Critical synaptic components are not expressed in larval secondary neurons, indicating that these neurons are immature, with no functional synapses. These include Rbp, encoding a presynaptic scaffold essential for neurotransmitter release, Sif, encoding a Rho-family GTPase involved in the reorganization of the actin cytoskeleton within the axonal terminals during synaptogenesis, Para, encoding an essential alpha subunit of the voltage-gated sodium channels, and Comt, encoding an ATPase required for the maintenance of neurotransmitter release. In the absence of any of these essential transcripts, there is no synaptic activity, either because synapses do not form properly or there is no action potential or sustained neurotransmitter release. Therefore, during larval stages, many adult neurons are born, acquire their cell identities, and differentiate, but halt their maturation until later developmental stages.
Most of these larval secondary neurons will mature as adult interneurons. In addition, the larval VNC employs a large population of mature, primary interneurons (born during the first wave of neurogenesis) that coordinate larval functions. We subclustered these primary interneurons into 48 clusters and found that each cluster was defined by unique combinations of transcription factors. Interestingly, the primary interneurons have very limited similarities with the secondary interneurons (born during the second wave of neurogenesis), which will function during adult stages. We reason that the limited similarities observed may primarily reflect differences between immature and actively connected neurons.
Our larval atlas offers unique insights into neurogenesis and into the strategies and signaling networks utilized for generation of the adult VNC. It also provides a high-resolution characterization of the larval VNC, capturing primary neurons, glia, and the functional landscape that coordinates larval behavior.
Versatile nanobody-based approach to image, track, and reconstitute functional synaptic proteins in vivo
Synaptic proteins, in particular the transmembrane proteins, are notoriously difficult to track and study because of their low abundance and high density of functional domains. Often, studies using transgenic lines that express selective tagged isoforms/variants cannot generate definitive conclusions because of heterologous promoters and overexpression artifacts. To facilitate structure-function studies and accomplish reliable detection of low-abundant synaptic proteins in different tissues, we turned to a recently described cell-biology tool, the ALFA system. The system consists of a synthetically designed epitope tag of only 14 amino acids, the ALFA tag (AT), with no homology in the animal kingdom, and a nanobody (NbALFA) that binds to ALFA–tagged proteins with picomolar affinities. The high affinity of ALFA tag/NbALFA binding and the intrabodies capabilities of NbALFA (that is binding ALFA–tagged proteins when expressed in living cells) have prompted the development of a variety of in vitro cell-biology applications, from super-resolution to live detection of tagged proteins.
To probe whether this methodology is suitable for in vivo application, we chose a case-study protein, Drosophila Neurexin-1 (Nrx-1). Neurexins are key adhesion proteins that coordinate extracellular and intracellular synaptic assembles. Neurexins are also very difficult to tag and study because of their low abundance, multiple extracellular functional modules, and intracellular protein-protein binding domains and docking motifs. The proteins are crucial for synapse assembly and function; however, the role of some of their domains (for example the C-terminal PDZ–binding motifs; PDZ domains are primarily involved in anchoring receptor proteins to the cytoskeleton) has been only inferred from in vitro studies. Guided by phylogenetic analysis and secondary structure prediction, we generated ALFA–tagged Nrx-1 variants, including an endogenously tagged Nrx-1AT allele, which is indistinguishable from the wild-type control, and a NrxdPDZ-AT allele that resembles the Nrx-1null mutant. Using a combination of classic genetics and cell biology and electrophysiology approaches, we found that Nrx-1AT NMJs have normal morphology and function, whereas the NrxdPDZ-AT mutants have smaller NMJs with much reduced basal neurotransmission, reminiscent of Nrx-1null mutant. Similar to untagged Nrx-1, endogenously edited Nrx-1AT localizes at presynaptic sites; remarkably, the ALFA system enabled detection of endogenous Nrx-1AT in only one immunohistochemistry step using the monovalent binder NbALFA conjugated to two fluorophores (FluoTag-X2 anti-ALFA). These data confirm the expectation that the ALFA system ensures high-affinity binding, linear (monovalent) signals with respect to target molecule, with no amplification by polyclonal secondaries, and virtually no background in animal tissues.
To test whether the ALFA system could facilitate compartment-specific detection of tagged proteins in vivo, we built genetically encoded NbALFA-mScarlet cytosolic chimera (UAS-Nb-mScarlet transgenes) and examined their subcellular distribution relative to ALFA–tagged and untagged Nrx-1. In the presence of untagged Nrx-1, Nb-mScarlet accumulated in the neuron soma; in the presence of Nrx-1-AT, Nb-mScarlet became restricted to near the neuronal membranes. This result indicates that the AT/NbALFA binding re-directs the cytoplasmic Nb-mScarlet to the synaptic terminals, where Nrx-1-AT resides. These multi-component complexes could also be followed in vivo, en route to the synaptic terminals: we detected mobile mScarlet–labeled vesicles in animals expressing UAS-Nrx-1-AT but not in untagged Nrx-1 controls. The estimated velocities of vesicles were 0.24± 0.03 mm/s (n=27) away from the ventral cord and 0.20± 0.10 mm/s (n=35) towards the ventral cord. Recent studies reported that Rab2–marked vesicles, originating from the trans-Golgi network, enable the trafficking of presynaptic components at the fly NMJ. Upon labeling the transport vesicles observed for Rab2, we found that Drosophila Nrx-1 co-migrates with Rab2–positive vesicles to synaptic terminals.
In addition, we found that the PDZ–binding motif is key to Nrx-1 in vivo surface expression and synaptic localization: the NrxdPDZ-AT variant was trapped in the ER, unable to traffic to the cell surface (Figure 4). This explains why the NrxdPDZ-AT allele had NMJ defects similar to the Nrx-1null mutant. Given that the ALFA system is very compact and has high binding affinity, both inside and outside the cells, we next asked whether this system could deliver the missing PDZ–binding motif in trans, facilitating the reconstitution of functional Nrx-1. To this end, we generated genetically encoded NbALFA-PDZ–binding motif chimera (UAS-Nb-PDZ) and expressed it in the NrxdPDZ-AT neurons. The resulting animals were viable and fertile and had normal NMJ morphology and function, indicating that a PDZ–binding motif provided in trans fully restored the synaptic localization and function of NrxdPDZ-AT.
The ability to use the ALFA system as a split system to reconstitute and track functional proteins in vivo opens up a new realm of possibilities for functional studies in specific cells/tissues and during defined developmental windows. We anticipate that the methodology will pave the way towards dissecting functional domains of complex proteins in vivo.
Figure 4. ALFA system as a versatile split-system for structure-function analyses of proteins in vivo
A. Domain organization of the Drosophila Nrx-1 with the ALFA-tag insertion site marked.
B–E. Confocal images of larval VNCs of the indicated genotypes labeled for ALFA tag (green) and Nrx-1 (magenta). The images were acquired with the same confocal microscopy settings and scaled equally for direct visual comparison. The edited Nrx-1AT shows the expected Nrx-1 distribution (C).
The absence of a PDZ–binding motif (domains that are primarily involved in anchoring receptor proteins to the cytoskeleton) disrupts the subcellular distribution of NrxdPDZ-AT (D). Addition of the PDZ–binding motif in trans restores the cell-surface location and normal synaptic distribution of reconstituted Nrx-1 (NrxdPDZ-AT/Nb-PDZ) (E).
F. Diagram for the in trans reconstitution of Nrx-1 (NrxdPDZ-AT + Nb-PDZ). The missing PDZ–binding motif of NrxdPDZ-AT is delivered in trans via genetically encoded NbALFA-PDZ–binding motif chimera (Nb-PDZ).
Publications
- Vicidomini R, Serpe M. Local BMP signaling: a sensor for synaptic activity that balances synapse growth and function. Curr Topics Dev Biol 2022 50:211–246.
- Nguyen TH, Vicidomini R, Choudhury S, Coon SL, Iben J, Brody T, Serpe M,. Single-cell RNA sequencing analysis of the Drosophila larval ventral cord. Curr Protoc 2021 e38.
- Vicidomini R, Nguyen TH, Choudhury S, Brody T, Serpe M. Assembly and exploration of a single cell atlas of the Drosophila larval ventral cord. Identification of rare cell types. Curr Protoc 2021 e37.
Collaborators
- Steven Coon, PhD, Molecular Genetics Core, NICHD, Bethesda, MD
- Fabio Faucz, PhD, Molecular Genetics Core, NICHD, Bethesda, MD
- James Iben, PhD, Molecular Genetics Core, NICHD, Bethesda, MD
- Dragan Maric, PhD, Flow and Imaging Cytometry Core, NINDS, Bethesda, MD
- Felipe Opazo, PhD, Center for Biostructural Imaging of Neurodegeneration, Göttingen, Germany
Contact
For more information, email mihaela.serpe@nih.gov or visit https://www.nichd.nih.gov/research/atNICHD/Investigators/Serpe.