Modeling the Biophysics of Cellular Membranes
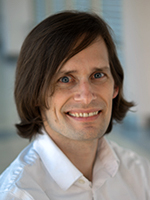
- Alexander J. Sodt, PhD, Head, Unit on Membrane Chemical Physics
- Andrew Beaven, PhD, Postdoctoral Fellow
- Amirali Hossein, PhD, Postdoctoral Fellow
- Kayla Sapp, PhD, Postdoctoral Fellow
- Noah Feng, BS, Postbaccalaureate Fellow
- Mayisha Nakib, G-SOAR (Graduate Summer Opportunity to Advance Research) Student
The integrity of lipid membranes is essential for life. They provide spatial separation of the chemical contents of the cell and thus make possible the electrical and chemical potential differences that are used to transmit signals and perform work. However, the membrane must be broken frequently to form, for example, new membrane structures in the cell. The simplest structure is a vesicle to transport cargo. Such vesicles are constantly cycled between organelles and the outer plasma membrane. Thus, there is a careful balance between boundary-establishing membrane fidelity and the necessary ability of the cell to change these boundaries.
The challenge in studying the membrane is its complexity. The membrane is a thin sheet of small molecules, i.e., lipids. There are hundreds of types of lipids in the cell. Each lipid changes the properties of the membrane in its vicinity, sometimes making the sheet stiffer, sometimes softer, and sometimes acting to bend the membrane into a ball or tube. Furthermore, the lipids are constantly jostling and tangling, both with each other and with proteins embedded in the membrane. To predict of how membranes are reshaped thus requires not only knowing how lipids affect the properties of the membrane surface, but also the location of specific lipids.
The question as to how molecular scale features influence extensive biological processes must be answered in the language of physical laws. Physics is the language of mechanism at the molecular scale. The challenge is linking physics to the ‘big’ processes that happen in life. Our lab uses detailed physics-driven molecular simulation to ‘build up’ models that can be applied at the much larger level of the cell, which requires retaining important information and eliminating irrelevant details. The software our lab develops is based on the models that we are building. Thus, a broad objective of our research is to create a publicly available software package that can be used either as a stand-alone application for analyzing membrane-reshaping processes or as a library for cellular-scale modeling packages for which the role of the membrane may be unclear or unanticipated.
Another key component of our research is to seek the best possible validation of our models. Few techniques are able to yield molecular information about lipids. Recent breakthroughs that break the diffraction-limit barrier are typically only applicable to static structures much larger than a molecular dye. In contrast, lipids are small and dynamic. Our group is making a sustained effort to validate our simulation findings by applying neutron scattering techniques. This year, our lab initiated new collaborations on the basis of our previous years' work developing methodology for predicting the scattering signal from our simulations.
The projects use the NIH computing resources, including the Biowulf cluster, to run simulations and models. We use molecular dynamics software (such as NAMD and CHARMM) to conduct molecular simulations. In-house software development for public distribution is a key element of the lab's work.
The unexpected influence of cholesterol on fusion/fission pore dynamics
Cellular membranes are the medium by which cellular protein machinery is transported to and from the plasma membrane. Patches of membrane are constantly reshaped by the cell into spherical transport vesicles. In this simulation study [Reference 1], we used a special-purpose-built computer resource (“Anton 2”) to test the effect of cholesterol dynamics on the shape and stability of membrane pores that are high-energy intermediates in the process of reshaping. We found that cholesterol is strongly excluded from the highly curved neck of the fusion pore. This is intuitively consistent with its tendency to thicken membranes, but inconsistent with its strong preference for the high curvature of the pore interior. We resolved this discrepancy with a modification to elasticity theory for cholesterol. In our model, cholesterol has a strong collapsing effect on fusion pores, favoring endocytosis. This may be one clue as to why cholesterol is enriched so strongly in the plasma membrane of human cells and what can go wrong in development if cholesterol levels are insufficient.
Membrane stiffness is strongly influenced by lipid lateral dynamics.
In a joint theory-experimental collaboration with the Dimova lab, we analyze the dynamic relaxation of giant unilamellar vesicles, a model system for biological membranes. We predicted that the relaxation timescales of mixtures of two lipids (with very different elastic properties) would be strongly influenced by slow diffusion, a prediction that was borne out in the experiment. The nanometer-scale implication is that lipids dynamically redistribute to regions of the membrane with shape that they favor, and thus, that mixtures of lipids nearly always tend to be softer than simpler bilayers. The membranes of living cells have hundreds of unique lipids, suggesting that lipid diversity is contributing to the unique mechanical properties of biological membranes.
Publications
- Beaven AH, Sapp K, Sodt AJ. Simulated dynamic cholesterol redistribution favors membrane fusion pore constriction. Biophys J 2023 122:2162–2175.
- Sapp K, Aleksanyan M, Kerr K, Dimova R, Sodt AJ. Kinetic relaxation of giant vesicles validates diffusional softening in a binary lipid mixture. Phys Rev E 2023 107(5–1):054403.
- Soubias O, Sodt AJ, Teague WE, Hines KG, Gawrisch K. Physiological changes in bilayer thickness induced by cholesterol control GPCR rhodopsin function. Biophys J 2023 122:973–983.
- Jimenez-Munguia I, Beaven AH, Blank PS, Sodt AJ, Zimmerberg J. Interferon-induced transmembrane protein 3 (IFITM3) and its antiviral activity. Curr Opin Struct Biol 2022 77:10246.
Collaborators
- Alex A. Compton, PhD, HIV Dynamics and Replication Program, Center for Cancer Research, NCI, Frederick, MD
- Rumiana Dimova, PhD, Max Planck Institute of Colloids and Interfaces, Potsdam, Germany
- Olivier Soubias, PhD, Structural Biophysics Laboratory, Center for Cancer Research, NCI, Frederick, MD
Contact
For more information, email alexander.sodt@nih.gov or visit https://www.nichd.nih.gov/research/atNICHD/Investigators/sodt.