Chemosensory Coding and Decoding by Neuron Ensembles
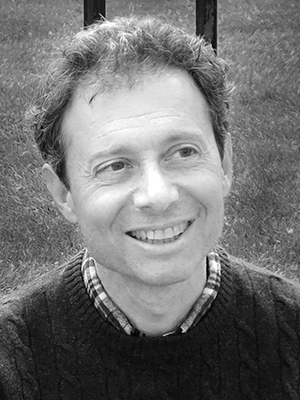
- Mark Stopfer, PhD, Head, Section on Sensory Coding and Neural Ensembles
- Zane Aldworth, PhD, Staff Scientist
- Yuzhong Cheng, PhD, Senior Research Assistant
- Kui Sun, MD, Research Assistant
- Alejandra Boronat Garcia, PhD, Postdoctoral Fellow
- Bo-Mi Song, PhD, Postdoctoral Fellow
- Brian Kim, BS, Graduate Student
- Leah Pappalardo, BS, Graduate Student
All animals need to know what is going on in the world around them. Brain mechanisms have thus evolved to gather and organize sensory information to build transient and sometimes enduring internal representations of the environment.
Using relatively simple animals and focusing primarily on olfaction and gustation, we combine electrophysiological, anatomical, behavioral, computational, optogenetic, and other techniques to examine the ways in which intact neural circuits, driven by sensory stimuli, process information. Our work reveals basic mechanisms by which sensory information is transformed, stabilized, and compared, as it makes its way through the nervous system.
We use three species of insects, each with specific and interlocking experimental advantages, as our experimental preparations: locusts, moths, and fruit flies. Compared with vertebrates, the insect nervous system contains relatively few neurons, most of which are readily accessible for electrophysiological study. Essentially intact insect preparations perform robustly following surgical manipulations, and insects can be trained to provide behavioral answers to questions about their perceptions and memories. Ongoing advances in genetics permit us to target specific neurons for optogenetic or electrophysiological recording or manipulations of activity. Furthermore, the relatively small neural networks of insects are ideal for tightly constrained computational models that test and explicate fundamental circuit properties.
Olfactory receptor neurons generate multiple response motifs, increasing coding space dimensionality.
Odors provide many types of important information about the environment and are characterized by their chemical compositions and concentrations. The tens or hundreds of thousands of detectable odorant molecules come in many different shapes, sizes, and charge distributions, requiring the brain to generate a high-dimensional description. Adding to this complexity, odorants often travel in chaotic and turbulent plumes comprising odorized pulses separated by clean air. The structures of odor pulses within a plume are determined by factors such as wind speed, environmental features such as hills, trees, or buildings, and distance from the source. Therefore, the timing of odorants reaching a sensor can convey important information about the surroundings. To make use of this information, olfactory systems must generate high-dimensional representations of information about an odorant’s chemical composition and delivery timing.
The process begins when odorants, binding to olfactory receptor neurons, trigger bursts of action potentials, providing the brain with its only experience of the olfactory environment. To better characterize the response dynamics of these neurons, we made hundreds of extracellular recordings from locust antennae in vivo, while presenting odor pulses individually or in regular trains, or as realistic, chaotic odor plumes. The recordings revealed something novel: rather than falling into a continuum of patterns, odor-elicited responses of olfactory receptor neurons clustered neatly into four distinct motifs, each defined by a reliable temporal profile that we called excitatory, delayed, offset, and inhibitory motifs. Different odorants could elicit different response motifs from a given olfactory receptor neuron, a novel property we termed motif switching. Further, each motif underwent its own form of sensory adaptation when activated by repeated odor pulses; for example, when repeatedly activated, excitatory responses significantly decreased, but offset responses significantly increased.
The response motifs we found in vivo seemed likely to contribute to the processing of olfactory information. To test this idea rigorously, we built a computational model based on our observations. Our model revealed that organizing responses into multiple motifs provides substantial benefits for classifying odors and processing complex odor plumes: each motif contributes uniquely to encode different aspects of the plume’s composition and structure. Multiple motifs and motif switching further improve odor classification by expanding the dimensionality of the coding space. Our model demonstrated that these response features could provide benefits for olfactory navigation, including measuring and encoding the distance to an odor source.
Our work provides a new perspective on the first stage of olfactory coding, revealing a fundamental way receptor neurons can begin the process of extracting many important features from a sensory stream.
Feedback inhibition and its control in an insect olfactory circuit
Inhibitory neurons play critical roles in regulating and shaping olfactory responses in vertebrates and invertebrates. In insects, these roles are performed by relatively few neurons, which can be interrogated efficiently, revealing fundamental principles of olfactory coding. GABAergic neurons play critical roles in mediating the sparsening of olfactory neural representations. With intracellular recordings and a new large-scale biophysical model, which includes tens of thousands of neurons and spans multiple layers of processing, we focus on the locust’s Giant GABAergic Neuron, GGN, to test ideas about how feedback inhibition creates a sparse odor representation in a higher order brain center.
Figure 1. Giant GABAergic neurons regulate olfactory responses in the locust brain.
The composite image shows the structure of a compartmental computational model of the giant GABAergic neurons (GGNs) superimposed on dextran-dyed mushroom bodies in the locust brain. Different branches of GGN are shown in different colors. GGNs, only one on each side of the brain, regulate the firing of tens of thousands of olfactory neurons through feedback inhibition.
The GGN’s giant structure is likely an important factor in its function. It receives excitatory input from all 50,000 Kenyon cells in the mushroom body’s α lobe and, in turn, provides inhibitory feedback to all Kenyon cells 400–500μm away in the calyx. The GGN is a huge neuron, but does not generate spikes, raising questions about how its far-flung regions interact. In addition to its large size, along its path from the α lobe to the calyx, its initially thick processes divide at myriad branch points into thin fibers. Cable theory applied to neurons predicts that a passive voltage signal within such a structure will attenuate dramatically. To test whether this giant neuron has the biophysical capacity to perform its suggested function of carrying effective signals passively from the α lobe to distant points in the calyx, we made dozens of recordings from the GGN in vivo, filled it with dye, and reconstructed it into a realistic computational model to characterize signal attenuation through its structure. Our model showed that, although electrical signals undergo substantial attenuation throughout its structure, signals in the GGN’s calyceal branches appear strong enough to provide global inhibition to Kenyon cells.
To further understand the network determinants of the GGN’s responses to odors, we recorded from it in vivo while delivering odors to the animal, and then used our large-scale model to investigate the types of network activity needed to generate the patterns we had observed in the GGN in vivo. We identified several novel features in the olfactory network. Surprisingly, our model predicted that a small portion of the notoriously quiet Kenyon cells must respond to odors with relatively high spike rates. We tested this prediction in vivo with patch-clamp recordings from many Kenyon cells while presenting odors to the animal’s antenna. Indeed, we confirmed that the predicted portion of hyperactive Kenyon cells really exists. We also documented novel, complex response patterns not previously observed in the GGN, including periods of hyperpolarization, that vary with the odorant. Our model predicts that this behavior is likely driven by a novel, yet unknown odor-activated pathway. Together, the results of our in vivo recordings and large-scale computational modeling provide a more complete understanding of how different parts of the olfactory system interact.
Identification and analysis of odorant receptors expressed in two main olfactory organs: antennae and palps of a model organism, the locust Schistocerca americana
Olfaction allows animals to detect, identify, and discriminate among hundreds of thousands of odor molecules present in the environment. It requires a complex process to generate the high dimensional neural representations needed to characterize odorant molecules, which have different sizes, shapes, and electrical charges, and are often organized into chaotic and turbulent odor plumes. Understanding the anatomical organization of the olfactory system at cellular and molecular levels has provided important insights into the coding mechanisms underlying olfaction, and studies performed in insects have contributed substantially to our knowledge of odor processing. Further, mechanisms that allow the olfactory system to generate representations for odors have been shown to be widely conserved among very divergent species. Extending our understanding of olfaction requires knowledge of the molecular and structural organization of the olfactory system.
Odor sensing begins with olfactory receptor neurons (ORNs), which express odorant receptors (ORs). In insects, ORNs are housed, in varying numbers, in olfactory sensilla. Because the organization of ORs within sensilla affects their function, it is essential to identify the ORs they contain. Using RNA sequencing, we identified 179 putative ORs in the transcriptomes of the two main olfactory organs, antenna and palp, of the locust Schistocerca americana. Quantitative expression analysis showed that most putative ORs (140) are expressed in antennae, while only 31 are in the palps. Further, one OR was detected only in palps, and seven are expressed differentially by sex. An in situ analysis of OR expression revealed at least six classes of sensilla in the antenna. A phylogenetic comparison of OR–predicted protein sequences revealed homologous relationships among two other Acrididae species. Our results provide a foundation for understanding the organization of the first stage of the olfactory system in S. americana, a well studied model for olfactory processing.
Development of the olfactory system
The sense of smell is essential for survival throughout the lifespan of most animals, from the moment of birth. Studying the olfactory system as an animal develops can reveal fundamental aspects of information processing by networks of neurons as they grow and interact with the environment. The study of relatively simple animals such as locusts has proved to be a useful strategy for answering basic questions about olfaction.
Many animals, including humans, display innate preferences for some odors, but the neural mechanisms underlying these preferences are poorly understood. To investigate the encoding of innate preferences, it is necessary to establish a model system that allows both clear behavioral demonstrations of olfactory preferences and tractable analyses of neural mechanism. Thus, using locusts that had just hatched, we designed and used an open-field arena to provide only olfactory cues to guide navigation choices, and we used our own Argos tracking software to quantify the movements of naïve hatchlings. We found that newly hatched locusts navigated toward, and spent more time near, the odor released by a typical locust food, wheat grass, than humidified air. Notably, in similar tests, we found that hatchlings avoided moderate concentrations of major individual components of the wheat grass odor, 1-hexanol and hexanal diluted in mineral oil relative to control presentations of unscented mineral oil. Hatchlings were neither attracted nor repelled by a lower concentration of 1-hexanol but were moderately attracted to a low concentration of hexanal.
Our behavioral results provide the first unambiguous demonstration that fresh-from-the-egg locust hatchlings have a strong, innate preference for the odor of wheat grass (a blend of many components), but the valence of the blend’s individual components may be different and may change depending on the concentration. Our results provide an essential entry point for an analysis of neural mechanisms underlying innate sensory preferences.
As locusts grow from hatchling to adulthood, they acquire experience with the olfactory environment, and their body size increases tenfold while their brain size almost triples. Understanding how these changes affect the processing of olfactory information requires a rigorous investigation of the structure and function of developing locusts.
We therefore conducted an analysis of the structure and function of the first stages in the olfactory pathway in newly hatched and adult locusts by making electrophysiological recordings from their neurons to characterize their responses to odors and filling them with dye to visualize their morphologies. Our quantitative comparisons indicate that, although smaller in size, hatchling olfactory neurons are functionally and structurally like those of adults. With electroantennograms elicited by a broad panel of odors, we determined that the tuning of the olfactory receptor neuron population is similar in hatchlings and adults. Furthermore, with patch-clamp recordings, we found that local and projection neurons of the antennal lobe respond to odors with quantitatively similar complex, synchronous, oscillatory activity patterns in hatchlings and adults.
Notably, we found that odor-elicited neural oscillations in hatchlings gradually increase in frequency as the locusts grow. Based on our earlier computational modeling results, we hypothesized that this frequency change could be explained by increased odor-elicited excitatory drive, which accrues as new olfactory receptor neurons are added to growing antennae. To test this hypothesis, we successively removed segments containing olfactory receptor neurons from the adult antenna while recording local field potentials from the mushroom bodies. Consistent with our hypothesis, removing antennal segments significantly reduced the frequency of odor-elicited neural oscillations only on the side of the brain ipsilateral to the clipped antenna.
Overall, our developmental analysis shows that locusts hatch with a fully formed olfactory system, which structurally and functionally resembles that of the adult, despite its small size and lack of prior experience with olfactory stimuli.
Spatiotemporal coding of individual chemicals by the gustatory system
Four of the five major sensory systems (vision, olfaction, somatosensation, and audition) are thought to be encoded by spatiotemporal patterns of neural activity. The exception is gustation. Gustatory coding by the nervous system is thought to be relatively simple, i.e., every chemical (‘tastant’) is associated with one of a small number of basic tastes, and the presence of a basic taste, rather than the specific tastant, is represented by the brain. In mammals as well as insects, five basic tastes are usually recognized: sweet, salty, sour, bitter, and umami. The neural mechanism for representing basic tastes is unclear. The most widely accepted postulate is that, in both mammals and insects, gustatory information is carried through labeled lines of cells sensitive to a single basic taste, that is, in separate channels from the periphery to sites deep in the brain. An alternative proposal is that the basic tastes are represented by populations of cells, with each cell sensitive to several basic tastes.
Testing these ideas requires determining, point-to-point, how tastes are initially represented within the population of receptor cells and how this representation is transformed as it moves to higher-order neurons. However, it has been highly challenging to deliver precisely timed tastants while recording cellular activity from directly connected cells at successive layers of the gustatory system. Using a new moth preparation, we designed a stimulus and recording system that allowed us to fully characterize the timing of tastant delivery and the dynamics of the tastant-elicited responses of gustatory receptor neurons and their monosynaptically connected second-order gustatory neurons, before, during, and after tastant delivery.
Surprisingly, we found no evidence consistent with a basic taste model of gustation. Instead, we found that the moth’s gustatory system represents individual tastant chemicals as spatiotemporal patterns of activity distributed across the population of gustatory receptor neurons. We further found that the representations are transformed substantially, given that many types of gustatory receptor neurons converge broadly upon follower neurons. The results of our physiological and behavioral experiments suggest that the gustatory system encodes information not about basic taste categories but rather about the identities of individual tastants. Furthermore, the information is carried not by labeled lines but rather by distributed, spatiotemporal activity, which is a fast and accurate code. The results provide a dramatically new view of taste processing.
Additional Funding
- NICHD Career Development Award to Bo-Mi Song
Publications
- Ray S, Aldworth Z, Stopfer M. Feedback inhibition and its control in an insect olfactory circuit. eLife 2020 9:e53281.
- Gupta N, Singh SS, Stopfer M. Oscillatory integration windows in neurons. Nat Commun 2016 7:13808.
- Reiter S, Campillo Rodriguez C, Sun K, Stopfer M. Spatiotemporal coding of individual chemicals by the gustatory system. J Neurosci 2015 35:12309–12321.
- Ray S, Stopfer M. Argos: a toolkit for tracking multiple animals in complex visual environments. Methods Ecol Evolution 2022 133:585–595.
- Aldworth ZN, Stopfer M. Insect neuroscience: filling the knowledge gap on gap junctions. Curr Biol 2022 32(9):R420–R423.
Collaborators
- Maxim Bazhenov, PhD, Howard Hughes Medical Institute, The Salk Institute for Biological Studies, La Jolla, CA
Contact
For more information, email stopferm@mail.nih.gov or visit https://www.nichd.nih.gov/research/atNICHD/Investigators/stopfer.