Studies on DNA Replication, Repair, and Mutagenesis in Eukaryotic and Prokaryotic Cells
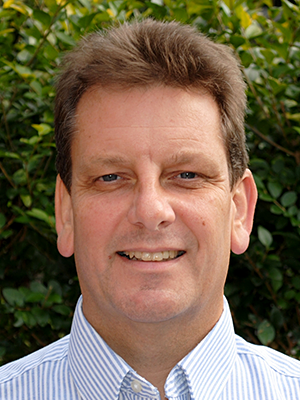
- Roger Woodgate, PhD, Chief, Section on DNA Replication, Repair, and Mutagenesis
- John P. McDonald, PhD, Biologist
- Mary McLenigan, BS, Chemist
- Nicholas W. Ashton, PhD, Postdoctoral Visiting Fellow
- Marcella T. Latancia, PhD, Postdoctoral Visiting Fellow
- Natalia C. Moreno, PhD, Postdoctoral Visiting Fellow
- Dana A. D'Orlando, BS, Postbaccalaureate Intramural Research Training Award Fellow
- Nickolas G. Moeckel, BS, Postbaccalaureate Intramural Research Training Award Fellow
- Samir Marwaha, Summer Student
- Jaan C. Selod, Summer Student
Under optimal conditions, the fidelity of DNA replication is extremely high. Indeed, it is estimated that, on average, only one error occurs for every 10 billion bases replicated. However, given that living organisms are continually subjected to a variety of endogenous and exogenous DNA–damaging agents, optimal conditions rarely prevail in vivo. While all organisms have evolved elaborate repair pathways to deal with such damage, the pathways rarely operate with 100% efficiency. Thus, persisting DNA lesions are replicated, but with much lower fidelity than in undamaged DNA. Our aim is to understand the molecular mechanisms by which mutations are introduced into damaged DNA. The process, commonly referred to as trans-lesion DNA synthesis (TLS), is facilitated by one or more members of the Y-family of DNA polymerases, which are conserved from bacteria to humans. Based on phylogenetic relationships, Y-family polymerases may be broadly classified into five subfamilies: DinB–like (pol IV/pol kappa–like) proteins are ubiquitous and found in all domains of life; in contrast, the Rev1–like, Rad30A (pol eta)–like, and Rad30B (pol iota)–like polymerases are found only in eukaryotes; and the UmuC (polV)–like polymerases only in prokaryotes. We continue to investigate TLS in all three domains of life: bacteria, archaea, and eukaryotes.
Prokaryotic studies
Strand specificity of ribonucleotide excision repair in E. coli
In Escherichia coli, replication of both strands of genomic DNA is carried out by a single replicase: DNA polymerase III holoenzyme (pol III HE). However, in certain genetic backgrounds, the low-fidelity TLS polymerase, DNA polymerase V (pol V), gains access to undamaged genomic DNA, where it promotes elevated levels of spontaneous mutagenesis preferentially on the lagging strand. As part of a collaboration with scientists at the Polish Academy of Sciences in Warsaw, Poland, we employed active-site mutants of pol III (pol III alpha_S759N) and pol V (pol V_Y11A) to analyze ribonucleotide incorporation and removal from the E. coli chromosome on a genome-wide scale under conditions of normal replication, as well as SOS induction. Using a variety of methods tuned to the specific properties of these polymerases (e.g., analysis of lacI mutational spectra, lacZ reversion assay, HydEn-seq, and alkaline gel electrophoresis), we presented evidence that repair of ribonucleotides from both DNA strands in E. coli is unequal. While RNase HII plays a primary role in leading-strand ribonucleotide excision repair (RER), the lagging strand is subject to other repair systems (RNase HI and, under conditions of SOS activation, also nucleotide excision repair). Importantly, we suggested that RNase HI activity can also influence the repair of single ribonucleotides incorporated by the replicase pol III HE into the lagging strand.
Identification of an inhibitor of LexA cleavage
As antibiotic resistance has become more prevalent, the social and economic impacts are increasingly pressing. Indeed, bacteria have developed the SOS response, which facilitates the evolution of resistance under genotoxic stress. The transcriptional repressor LexA plays a key role in this response. Mutation of LexA to a non-cleavable form that prevents the induction of the SOS response sensitizes bacteria to antibiotics. Achieving the same inhibition of proteolysis with small molecules also increases antibiotic susceptibility and reduces acquisition of drug resistance. Previous attempts to develop inhibitors have investigated 1,2,3-triazole molecules binding to the hydrophobic cleft, and boronic acids that covalently bound to Ser-119. Neither of these resulted in any molecules progressing to preclinical trials. In collaboration with scientists at the Queensland Institute of Technology in Brisbane, Australia, we found that the cleavage site region (CSR) of the LexA protein is a classical Type II beta-turn, and that published 1,2,3-triazole compounds mimic the beta-turn. Based on this, we took a dual approach to the identification of a novel proteolytic inhibitor. We docked generic covalent molecule libraries and a β-turn–mimetic library to the LexA C-terminal domain using molecular modelling methods in FlexX and CovDock. The 133 highest scoring molecules were screened for their ability to inhibit LexA cleavage under alkaline conditions and the top molecules were then tested using a RecA–mediated counter assay, research that led to the discovery of an electrophilic serine warhead that can inhibit LexA proteolysis, reacting with Ser-119 via a nitrile moiety. Our studies therefore present a starting point for hit-to-lead optimization, which could lead to inhibition of the SOS response and prevent the acquisition of antibiotic resistance.
Characterization of the mycobacterial mutasome
A DNA damage–inducible mutagenic gene cassette has been implicated in the emergence of drug resistance in Mycobacterium tuberculosis during anti-tuberculosis (TB) chemotherapy. However, the molecular composition and operation of the encoded “mycobacterial mutasome,” minimally comprising DnaE2 polymerase and ImuA′ and ImuB accessory proteins, remain elusive. As part of a large international collaboration led by our collaborator Digby Warner, we exposed mycobacteria to DNA–damaging agents and observed that DnaE2 and ImuB co-localize with the DNA polymerase III beta subunit (beta clamp) in distinct intracellular foci. Notably, genetic inactivation of the mutasome in an imuB mutant containing a disrupted beta clamp–binding motif abolishes ImuB–beta clamp focus formation, a phenotype recapitulated pharmacologically by treating bacilli with griselimycin, as well as in biochemical assays in which this beta clamp–binding antibiotic collapses pre-formed ImuB–beta clamp complexes. These observations established the essentiality of the ImuB–beta clamp interaction for mutagenic DNA repair in mycobacteria and identified the mutasome as a target for adjunctive therapeutics designed to protect anti–TB drugs against emerging resistance.
Publications
- Łazowski K, Faraz M, Vaisman A, Ashton NW, Jonczyk P, Fijalkowska IJ, Clausen AR, Woodgate R, Makiela-Dzbenska K. Strand specificity of ribonucleotide excision repair in Escherichia coli. Nucleic Acids Res 2023 51(4):1766–1782.
- Schuurs ZP, McDonald JP, Croft LV, Richard DJ, Woodgate R, Gandhi NS. Integration of molecular modelling and in vitro studies to inhibit LexA proteolysis. Front Cell Infect Microbiol 2023 13:1051602.
- Gessner S, Martin ZA, Reiche MA, Santos JA, Dinkele R, Ramudzuli A, Dhar N, de Wet TJ, Anoosheh S, Lang DM, Arron J, Chew TL, Herrmann J, Müller R, McKinney JD, Woodgate R, Mizrahi V, Venclovas Č, Lamers MH, Warner DF. The mycobacterial ImuA'-ImuB-DnaE2 mutasome: composition and recruitment in live cells. Elife 2023 12:e75628.
Collaborators
- Irina Bezsonova, PhD, University of Connecticut, Farmington, CT
- Anders R. Clausen, PhD, Göteborgs Universitet, Göteborg, Sweden
- Michael Cox, PhD, University of Wisconsin, Madison, WI
- Tom C. Evans, PhD, New England Biolabs, Ipswich, MA
- Iwona Fijlakowska, PhD, Polish Academy of Sciences, Warsaw, Poland
- Neha S. Ghandi, PhD, Queensland University of Technology, Brisbane, Australia
- Martín Gonzalez, PhD, Southwestern University, Georgetown, TX
- Myron F. Goodman, PhD, University of Southern California, Los Angeles, CA
- Karolina Makiela-Dzbenska, PhD, Polish Academy of Sciences, Warsaw, Poland
- Justyna McIntyre, PhD, Polish Academy of Sciences, Warsaw, Poland
- Andrew Robinson, PhD, University of Wollongong, Wollongong, Australia
- Ewa Sledziewska-Gojska, PhD, Polish Academy of Sciences, Warsaw, Poland
- Antoine Van Oijen, PhD, University of Wollongong, Wollongong, Australia
- Digby Warner, PhD, University of Cape Town, Cape Town, South Africa
- Wei Yang, PhD, Laboratory of Molecular Biology, NIDDK, Bethesda, MD
Contact
For more information, email woodgate@.nih.gov or visit https://www.nichd.nih.gov/research/atNICHD/Investigators/woodgate.