You are here: Home > Molecular Neurophysiology and Biophysics Unit
Potassium Channels and Dendritic Function in Hippocampal Pyramidal Neurons
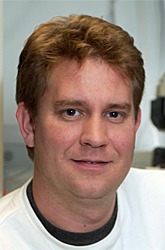
- Dax Hoffman, PhD, Head, Molecular Neurophysiology and Biophysics Unit
- Begum Choudhury, MS, Biologist
- Sung-Cherl Jung, PhD, Visiting Fellow
- Eunyoung Kim, PhD, Postdoctoral Fellow
- Lin Lin, PhD, Research Fellow
- Michael W. Nestor, PhD, Postdoctoral Fellow
- Wei Sun, BS, Graduate Student
The central nervous system underlies all our experiences, actions, emotions, knowledge, and memories. With billions of neurons each firing hundreds of times per second, the complexity of the brain is stunning. To pare down such complexity, our research approach calls for studying the workings of a single central neuron—the pyramidal neuron from the CA1 region of the hippocampus, a region of the brain important for learning and memory and among the first affected in Alzheimer’s disease and epilepsy. In the dendrites of hippocampal CA1 pyramidal neurons, a nonuniform density of subthreshold, rapidly inactivating potassium channels regulates signal propagation. With higher expression in the dendrites than in the soma, this distribution means that the electrical properties of the dendrites differ markedly from those of the soma. Incoming synaptic signals are shaped by the activity of these channels, and, once initiated in the axon, action potentials (AP) progressively decrease in amplitude as they propagate back into the dendrites. Combining patch clamp recording with molecular biology and imaging, we investigate the electrophysiological properties and molecular nature of the voltage-gated channels expressed in CA1 dendrites, how their expression is regulated, and their role in learning and memory.
Kv4.2 control of firing patterns in hippocampal CA1 pyramidal neurons
Although recent molecular cloning studies have found several families of voltage-gated K+ channel genes expressed in the mammalian brain, information about the relationship between the protein products of these genes and their various neuronal functions is currently lacking. We have used a combination of molecular, electrophysiological, and imaging techniques to show that the voltage-gated potassium channel subunit Kv4.2 controls AP half-width, frequency-dependent AP broadening, and dendritic AP propagation. To visualize Kv4.2, we fused the enhanced green fluorescence protein (EGFP) to the cytoplasmic C-terminus (Kv4.2g). Using a Sindbis virus, modified such that cytotoxicity is reduced and neuro-specificity of infection enhanced, we expressed either Kv4.2g or an EGFP-tagged dominant negative mutant of Kv4.2 [Kv4.2g(W362F)] in dissociated hippocampal neurons and in CA1 pyramidal neurons of organotypic slice cultures. Others have shown that Kv4.2g(W362F) acts as a subfamily-specific dominant negative (i.e., blocking only Kv4.x or shal-containing channels after heterologous expression).
Nucleated and outside-out patch clamp recordings from CA1 hippocampal neurons expressing Kv4.2g showed a two-fold higher transient outward current density than in non-expressing control neurons without an effect on the sustained component density (Kim et al., J Physiol 2005;569:41). Expression of the dominant negative Kv4 mutation knocked down the transient outward current density to 63% of control, again without affecting the sustained outward current density. Kv4.2g and Kv4.2g(W362F) expression did not alter transient current steady-state activation and inactivation. A comparison of Kv4.2g properties expressed in HEK-293 cells and in CA1 neurons suggests that an auxiliary subunit modulates Kv4.2g channel properties in neurons. In CA1 neurons, all measured kinetics (time-to-peak, inactivation rate, and rate of recovery from inactivation) are greater than those recorded in HEK-293 cells. These differences can be attributed to the auxiliary protein DPPX. We have started to characterize the functional role of DPPX in CA1 neurons (see below).
To assess the contribution of Kv4 family subunits to the electrical properties and firing patterns of CA1 pyramidal neurons, we performed whole-cell current-clamp experiments in each of the three experimental groups (EGFP, Kv4.2g, and Kv4.2g(W362F)). Suprathreshold current injections in Kv4.2g– and Kv4.2g(W362F)–expressing neurons showed that Kv4.2 is engaged throughout the physiologically relevant voltage range, contrary to the established view of somatic A-type currents acting solely subthreshold. In response to depolarization, Kv4.2 prevented AP initiation by delaying AP onset and increasing the threshold for initiation. We found that, subsequent to initiation, Kv4.2 aids in repolarization and contributes to the fast after-hyperpolarization potential. Single AP half-width was greatly reduced in Kv4.2g–expressing neurons, and somatic Ca2+ influx was barely half that of control neurons. Accumulating Kv4.2 inactivation during an AP train contributed to frequency-dependent AP broadening and subsequent somatodendritic Ca2+ influx. Finally, Kv4.2g limited backpropagation, as measured with Ca2+ imaging, whereas Kv4.2g(W362F) enhanced propagation.
The results show a prominent role for Kv4.2 in regulating AP shape and dendritic signaling. As Ca2+ influx occurs primarily during AP repolarization, Kv4.2 activity can regulate cellular processes involving Ca2+-dependent second messenger cascades such as gene expression and synaptic plasticity. We plan to extend our studies to include dendritic recordings of back-propagating APs in acute slice from adult mice after in vivo viral injections.
Kv4.2 trafficking in CA1 pyramidal neuron dendrites
Using a modified Sindbis virus system to overexpress EGFP–labeled Kv4.2 (Kv4.2g) in cultured hippocampal neurons, we found that the EGFP fluorescence in dendritic spines of Kv4.2g-expressing neurons appeared brighter than that from the adjacent dendritic shaft. The ratio of spine-head to dendritic-shaft fluorescence in Kv4.2g-expressing neurons was approximately two-fold greater than in neurons expressing EGFP. In collaboration with Ron Petralia, we further demonstrated Kv4.2 expression in spines with electronmicroscopy.
We found that stimulation (AMPA) resulted in an activity-dependent redistribution of Kv4.2g away from spines to the dendritic shaft and a punctate accumulation of Kv4.2g within the soma. This AMPA–induced redistribution of Kv4.2g occurred within 15 minutes of stimulation and was reversible, indicating that the treatment was not excitotoxic. Co-expression with pre- and postsynaptic markers showed that Kv4.2 undergoes activity-induced redistribution without a gross change in synaptic architecture or number. We confirmed these findings with live imaging of Kv4.2g removal from the spine in response to AMPA stimulation. We directly measured the effect of internalization as a decrease in the endogenous whole-cell transient K+ current from uninfected hippocampal neurons without a change in sustained or non-inactivating delayed rectifier-type voltage-gated K+ current amplitudes. Thus, activity-dependent Kv4.2 internalization occurs natively and is not an artifact of overexpression.
More recently, we examined the role of protein kinase A (PKA) in Kv4.2 activity–dependent trafficking. In hippocampal neurons, PKA activation with forskolin or 8-Br-cAMP induced Kv4.2 internalization from dendritic spines, whereas PKA inhibition prevented AMPA–induced internalization. Furthermore, introduction of a point mutation at the C-terminal PKA phosphorylation site of Kv4.2 (S552A) prevented the AMPA–induced internalization of Kv4.2. Together, the data demonstrate that activity-dependent internalization of Kv4.2 requires PKA phosphorylation of Kv4.2 at S522. We also examined the role of the Kv4.2 C-terminal dileucine internalization motif in PKA-mediated Kv4.2 internalization. The dileucine motif on Kv4.2 is important for dendritic targeting of Kv4.2 but does not affect the basal rate of Kv4.2 endocytosis. To determine whether the Kv4.2 dileucine motif mediates activity-dependent Kv4.2 internalization, we mutated the Kv4.2 C-terminal dileucine residues 481 to 482 to alanines (Kv4.2LLAA). When expressed in COS7 cells, Kv4.2g but not Kv4.2LLAA is internalized after forskolin application. The residues are therefore likely to mediate activity-dependent internalization of Kv4.2. We continue to investigate the mechanisms of Kv4.2 expression and trafficking.
Role of voltage-gated potassium channels in synaptic and intrinsic plasticity
For several decades, synaptic plasticity has been considered the best candidate mechanism for the formation and storage of memories. However, efficacy in driving a neuron to fire is dependent not only on the size of synaptic input but also on its location and timing, which is subsequently shaped by types and distributions of voltage- and calcium-gated conductances in dendrites. Several studies, including the original description of long-term potentiation (LTP) (Bliss and Lomo, J. Physiol 1973;232:331), have shown that the induction of synaptic plasticity is accompanied by changes in the intrinsic excitability of the neuron, indicating a potential concurrent change in voltage-gated channel activity. Activity-dependent regulation of intrinsic excitability (intrinsic plasticity), which is induced by learning, has been observed in several preparations. Plasticity in voltage- and/or calcium-gated channel function may then contribute to the memory process.
Using the Sindbis virus system to infect organotypic slice cultures with Kv4.2g and Kv4.2g(W362F), we have begun investigating the role of Kv4.2 in synaptic LTP, a potential mechanism for learning and memory. For the first 10 minutes after virus-mediated induction, potentiation is similar in all three groups—control, Kv4.2g, and Kv4.2g(W362F)—achieving about a 100% increase in excitatory postsynaptic current (EPSC) size. After this period, however, Kv4.2–overexpressing neurons fail to maintain potentiation, such that EPSC size returns to baseline after about 25 minutes. Conversely, expression of Kv4.2g(W362F) results in a potentiation that reaches a greater level 40 to 50 minutes after initiation than do controls. The data indicate that the functional expression level of Kv4.2 influences the degree of potentiation. We found that altering the functional Kv4.2 expression level also leads to a rapid, bidirectional remodeling of CA1 synapses. Neurons exhibiting enhanced A-type K+ current (IA) showed a decrease in relative synaptic NR2B/NR2A subunit composition and, as noted, did not exhibit LTP. Conversely, reducing IA by expression of a Kv4.2 dominant negative or through genomic knockout of Kv4.2 led to an increased fraction of synaptic NR2B/NR2A and enhanced LTP. Bidirectional synaptic remodeling was mimicked in experiments manipulating intracellular Ca2+ and was dependent on spontaneous activation of NMDA receptors and active CaMKII. Our data suggest that A-type K+ channels are an integral part of a synaptic complex that regulates Ca2+ signaling through spontaneous NMDAR activation to control synaptic NMDAR expression and plasticity. We are currently characterizing the mechanisms of Kv4.2’s effect on NMDARs and LTP.
We have found that LTP induction results in a rapid, long-lasting increase in intrinsic excitability of CA1 pyramidal neurons from organotypic slice cultures. While several channels may be modulated by protocols used to induce synaptic plasticity and thus lead to enhanced intrinsic excitability, A-type K+ channels, with a subthreshold activation range, are among the first channels activated upon depolarization, thereby determining AP onset time, threshold, and interspike intervals (Storm, Prog Brain Res 1990;83:161). The significant decreases in AP threshold, onset time, and AHP after LTP are all consistent with A-current downregulation (Kim et al., J Physiol 2005;569:41). To measure changes in A-current density and properties after LTP, Sung-Cherl Jung pulled nucleated patches from CA1 somata in organotypic slice cultures before and then every 10 minutes after LTP induction by a depolarization-pairing protocol. We then compared the current density values obtained with those found for neurons undergoing depolarization but no pairing (unpaired). Results show a clear, substantial (about 15 mV) negative inactivation curve shift in A-current density 10 minutes after pairing with a return to control values by 30 minutes after induction. In addition, our results indicate that Kv4.2 is internalized upon LTP induction. We observed no lasting change in sustained current density. The time course of the decrease in A-current matches well that shown previously after chemical LTP induction. We propose that the long-lasting increase in excitability induced with LTP occurs through the two-phase decrease in A-current activity: an immediate but short-lived (about 10 minutes) hyperpolarized shift in the voltage dependence of the steady-state inactivation curve of A-type K+ currents followed by a progressive, long-term decrease in peak A-type K+ current amplitude that outlasts the observed A-channel inactivation curve shift. One outcome of A-channel internalization after LTP is a decreased AP threshold, a global enhancement of EPSP-spike coupling.
Role of auxiliary proteins in regulating Kv4.2 expression and function
A-type K+ currents have unique kinetic and voltage-dependent properties that allow them to fine-tune synaptic events, APs, and neuronal firing. However, Kv4 currents in heterologous cells display slower kinetics of inactivation and recovery from inactivation than that typically recorded in neurons. Moreover, Kv4.2 channels likely make up the majority of the A-type current in several cell types, including hippocampal CA1 pyramidal neurons, layer 5 cortical neurons, and granule cells of the olfactory bulb, yet A-current properties and distributions vary between these cell types. Reconciliation of these properties came with the finding of neuron-specific auxiliary subunit expression of KChIPs and DPLs. In hippocampal CA1 pyramidal neurons, DPPX (also called DPP6) is the prominent DPL family member while two distinct KChIPs (KChIP2 and KChIP4) are expressed. When Kv4 subunits are co-expressed with DPPX, many properties of native CA1 A-type currents are restored.
To investigate the physiological role of DPPX in CA1 neurons, we developed, in collaboration with Bernardo Rudy’s laboratory, short interfering RNAs (siRNAs) to suppress the expression of all DPPX variants. We tested the efficacy of the siRNAs in CHO cells co-expressing Kv4.2 and DPPX-S. The reduction of DPPX proteins in CHO cells transfected with DPPX siRNA (siDPPX) was more than 95% complete, as quantified by immunoblotting. To investigate whether DPPX alters the kinetics of A-type currents in a native system, we conducted voltage-clamp experiments in outside-out patches from CA1 pyramidal neurons in hippocampal organotypic slices infected with siDPPX, using the Sindbis virus system. After allowing 2 to 3 days post-infection for DPPX knockdown, we found, in accordance with heterologous studies, that siDPPX results in a delayed recovery from inactivation, a slowed time-to-peak, and a rightward shift in the steady-state inactivation and activation curves for A-type currents.
To determine the physiological effect of the A-type current kinetic modifications by siDPPX, we carried out current-clamp experiments in siDPPX–expressing cells. Compared with negative control siRNA–expressing neurons, siDPPX-infected neurons exhibited lower input resistance, delayed time to AP onset, higher AP threshold, lower firing frequency, greater AP half-width, and lower fast AHP amplitudes. Thus, siDPPX had contrasting effects; it decreased the excitability subthreshold and increased the excitability suprathreshold. We used computer modeling to determine which of these sub- and suprathreshold effects may be explained by the shift in inactivation and activation curves. In addition, we are now investigating the role of DPPX in synaptic integration and plasticity in CA1 dendrites from knockout mice created in the Rudy laboratory.
Publications
- Hammond RS, Lin L, Sidorov MS, Wikenheiser AM, Hoffman DA. Protein Kinase A mediates activity-dependent Kv4.2 channel trafficking. J Neurosci 2008 28:7513-7519.
- Jung SC, Kim J, Hoffman DA. Rapid, bidirectional remodeling of synaptic NMDA receptor subunit composition by Atype K+ channel activity in hippocampal CA1 pyramidal neurons. Neuron 2008 60:657-671.
- Kim J, Hoffman DA. Potassium channels: newly found players in synaptic plasticity. Neuroscientist 2008 14:276-286.
- Kim J, Nadal MS, Clemens AM, Baron M, Jung SC, Misumi Y, Rudy B, Hoffman DA. The Kv4 accessory protein DPPX is a critical regulator of membrane excitability in hippocampal CA1 pyramidal neurons. J Neurophysiol 2008 100:1835-1847.
- Jung SC, Hoffman DA. Biphasic somatic A-type K channel downregulation mediates intrinsic plasticity in hippocampal CA1 pyramidal neurons. PLoS 2009 4(8):e6549.
Collaborator
- Bernardo Rudy, MD, PhD, New York University Medical Center, New York, NY
Contact
For more information, email buonanno@mail.nih.gov or visit http://neuroscience.nih.gov/Lab.asp?Org_ID=480.