You are here: Home > Section on Cell Cycle Regulation
Mitotic Regulation in Higher Eukaryotes by Ran and SUMO-1
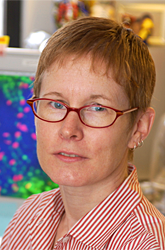
- Mary Dasso, PhD, Head, Section on Cell Cycle Regulation
- Alexei Arnaoutov, PhD, Visiting Fellow
- Maiko Furuta, PhD, Visiting Fellow
- Maria Lianguzova, PhD, Visiting Fellow
- Kara Lukasiewicz, PhD, Postdoctoral Fellow
- Ram Kumar Mishra, PhD, Visiting Fellow
- Debaditaya Mukhopadhyay, PhD, Visiting Fellow
- Maia Ouspenskaia, DVM, Biologist
- Younggang Wang, PhD, Visiting Fellow
- Hyun-Joo Yoon, PhD, Visiting Fellow
Our studies focus on two closely linked biochemical pathways that have been implicated in both mitotic regulation and nuclear-cytoplasmic trafficking: the SUMO pathway and the Ran pathway. SUMO proteins are a family of ubiquitin-like proteins that become covalently conjugated to cellular targets. Our studies on SUMOylation investigate the physiological roles of ubiquitin-like protease/sentrin–specific protease (Ulp/SENP) family members. Ulps/SENPs play a pivotal role in determining the spectrum of conjugated species by directly regulating the production of free, conjugatable SUMO proteins and the half-life of conjugated species. The Ran GTPase controls many cellular functions, including nucleocytoplasmic trafficking, spindle assembly, nuclear assembly, and cell cycle progression. We are particularly interested in the role(s) of Ran at mitotic kinetochores, where it is essential for regulation of the spindle assembly checkpoint and for assembly of microtubule fibers that attach kinetochores to spindle poles. We are currently focusing on mechanisms that target Ran pathway components to the kinetochore as well as on interactions of Ran pathway components at kinetochores with other proteins that are structural or functional components of the interphase nuclear pore.
SUMO family small ubiquitin-like modifiers in higher eukaryotes
SUMO proteins are a family of ubiquitin-related proteins that become covalently linked to other cellular proteins. Human SUMO proteins have been implicated in a variety of cell functions, including nuclear trafficking, chromosome segregation, chromatin organization, transcription, and RNA metabolism. There are three commonly expressed mammalian SUMO paralogs, called SUMO-1, SUMO-2, and SUMO-3. SUMO-2 and SUMO-3 are 96% identical, while SUMO-1 is roughly 45% identical to either SUMO-2 or SUMO-3. In this report, SUMO-2 and SUMO-3 are collectively referred to as SUMO-2/3. SUMO-1 is less abundant than SUMO-2/3, is conjugated to a distinct spectrum of targets, and has different in vivo dynamics and responses to physiological stress such as heat shock. Unlike SUMO-2/3, SUMO-1 is concentrated at nuclear pore complexes (NPCs), the primary conduit of nucleocytoplasmic trafficking, reflecting the fact that it is the preferred conjugation partner of RanGAP1. RanGAP1 is the GTPase activator for Ran, a small GTPase that controls nuclear transport. SUMO-1 conjugation of RanGAP1 promotes its stable association to the NPCs through binding to a large nucleoporin, RanBP2.
The conjugation pathway for SUMO proteins is similar to the ubiquitin conjugation pathway: SUMO proteins are processed by Ulps/SENPs to reveal a di-glycine motif at their C-termini. After processing, SUMO proteins undergo ATP-dependent formation of a thioester bond to their activating (E1) enzyme, Aos1/Uba2. The activated SUMO proteins are transferred to form a thioester linkage with their conjugating (E2) enzyme, Ubc9. Finally, an isopeptide bond is formed between SUMO proteins and substrates through the cooperative action of Ubc9 and protein ligases (E3). The linkage of SUMO proteins to their substrates can be severed by Ulps/SENPs, so it is likely that SUMO modification is highly dynamic in vivo. Ulp/SENPs play an important role in determining the spectrum of conjugated species because they directly regulate the production of free, conjugatable SUMO proteins and the half-life of conjugated species. There are six members of the Ulp/SENP family in mammals and five in amphibians (Xenopus laevis). We are systematically evaluating the physiological roles and regulation of these enzymes.
To investigate how Ulp/SENPs are regulated in a developmental context, we isolated and characterized all Ulp/SENPs in Xenopus laevis. Xenopus possesses homologs of mammalian SENP3, SENP5, SENP6, and SENP7. All these enzymes reacted with HA-tagged vinyl sulfone derivatives of SUMO-2 (HA-SU2-VS) but not of SUMO-1 (HA-SU1-VS), suggesting that the enzymes act primarily on SUMO-2/3. In contrast, Xenopus possesses a single member of the SENP1/SENP2 subfamily of Ulp/SENPs, most closely related to mammalian SENP1. Xenopus SENP1 reacted with HA-SU1-VS and HA-SU2-VS, suggesting that it acts on all SUMO paralogs. We analyzed the mRNA and protein levels for each Ulp/SENP through development; we found that they show distinct patterns of expression that may involve both transcriptional and post-transcriptional regulation. Lastly, we characterized the developmental function of SENP3, the most abundant Ulp/SENP found within Xenopus eggs. Depletion of SENP3 using morpholino antisense oligonucleotides (morpholinos) caused accumulation of high molecular weight SUMO-2/3–conjugated species, defects in developing embryos, and changes in the expression of some genes regulated by the transforming growth factor beta pathway. These findings collectively indicate that SUMO proteases are both highly regulated and essential for normal development.
We analyzed the physiological role of SENP3 in greater detail using both mammalian cells and Xenopus egg extracts. We found that SENP3 binds stably to a set of ribosome assembly factors that are homologous to the yeast Rix1 complex. SENP3 and the associated Rix1-complex components localize within the granular component of the nucleolus, a sub-nucleolar compartment that contains B23/Nucleophosmin. B23/Nucleophosmin is present in the extract under both high and low Ran-GTP conditions—it is not bound to the SENP3-Rix1 complex under low Ran-GTP conditions, while RanBP5 binds instead. When RanGTP levels are elevated, the situation is reversed: B23/Nucleophosmin binds to SENP3-Rix1, and RanBP5 is released. (It is attractive to speculate that either B23/Nucleophosmin or RanBP5 might physically displace the other, but we have not been able to show such a relationship using purified components.) Moreover, depletion of SENP3 or Rix1 complex members causes defects in ribosome biogenesis reminiscent of phenotypes observed in the absence of B23/Nucleophosmin. Together, our findings demonstrate striking conservation between the ribosome biogenesis pathways in yeast and vertebrates and suggest that regulation of SUMO deconjugation may be a major facet of B23/Nucleophosmin function in vivo. In addition, they provide a novel mechanism through which a Ran-regulated switch could control late stages of ribosome assembly.
We had previously shown that SENP6 (also called SUSP1) localizes within the nucleoplasm, where it plays a specialized role in dismantling highly conjugated SUMO-2/3 species. It was recently shown that poly-SUMO-2/3–conjugated species are frequently degraded through the action of RNF4, an ubiquitin ligase that targets them for proteasomal degradation. HeLa cells lacking SENP6 showed defects in spindle assembly and metaphase chromosome congression. Analysis of kinetochore composition in these cells revealed that a subset of proteins became undetectable on inner kinetochores after SENP6 depletion, particularly the CENP-H/I/K complex, whereas other changes in kinetochore composition mimicked defects previously reported to result from CENP-H/I/K depletion. We further found that CENP-I is degraded through the action of RNF4, and that SENP6 stabilizes CENP-I by antagonizing RNF4. Together, these findings reveal a novel mechanism whereby the finely balanced activities of SENP6 and RNF4 control vertebrate kinetochore assembly through SUMO-targeted destabilization of inner plate components.
Regulation of mitotic kinetochores by the Ran GTPase
We previously showed that Ran has two important roles at mitotic kinetochores. First, it is essential for the regulation of the spindle assembly checkpoint (SAC). The SAC is a cell cycle–regulatory pathway that prevents the onset of anaphase until all chromosomes are properly attached to the mitotic spindle and aligned on the metaphase plate. SAC accomplishes this task by monitoring the attachment of spindle microtubules to kinetochores—proteinaceous structures that assemble at the centromeric regions of each sister chromatid during mitosis. Failure of SAC is associated with chromosomal instability (CIN) leading to an abnormal chromosome number (aneuploidy). Second, Ran is essential for assembly of microtubule (MT) fibers that attach kinetochores to spindle poles (k-fibers). We showed that RanBP2, a large Ran-binding nucleoporin, and RanGAP1, the GTPase-activating protein for Ran, are targeted to kinetochores during mitosis as a single complex, which is both regulated by and important for stable kinetochore–MT association in mitotic spindles. We further found that Crm1, a Ran-GTP–binding nuclear export receptor, localizes to kinetochores in mammalian cells.
We are currently working on two aspects of Ran function at kinetochores. First, we are attempting to identify the effector for Ran that mediates its action on kinetochore-bound SAC proteins. We previously showed that elevated levels of Ran–GTP abrogate SAC-mediated mitotic arrest in Xenopus egg extracts (XEEs) and disrupt the kinetochore localization of SAC components, suggesting that the SAC is directly responsive to the overall concentration of Ran–GTP in that system. Our data strongly suggest that these effects are not mediated by known mitotic Ran effectors. We are currently examining other known Ran–GTP binding proteins, particularly nuclear transport receptors (karyopherins), to determine how they may contribute to SAC activation, and we are designing assays to identify novel components of this pathway.
Second, we are investigating how Crm1 promotes k-fiber assembly. We found that Crm1 localizes to kinetochores and that inhibition of Crm1 ternary complex formation by the inhibitor Leptomycin B (LMB) blocks kinetochore recruitment of RanGAP1/RanBP2. Crm1 itself requires neither ternary complex assembly nor MTs for kinetochore binding. Our findings support a direct role of the RanGAP1/RanBP2 in correct k-fiber assembly and suggest that Ran has a kinetochore-associated effector pathway that can be clearly differentiated from Importin-beta–mediated inhibition of soluble spindle assembly factors. The component(s) at kinetochores that is(are) directly involved in Crm1 recruitment is a major focus of our ongoing studies, which include both investigation of Ran-dependent interactions of Crm1 with kinetochore proteins and screens to identify mitotic-specific cargos that may be controlled through Crm1 binding.
Mitotic roles of nuclear pore complex (NPC) proteins
Vertebrate NPCs are large structures, with an estimated mass of 125 MD. NPCs contain about 30 distinct polypeptides, and 18 additional proteins have been classified as NPC-associated proteins. During interphase, NPCs are conduits for the trafficking of molecules between the cytoplasm and nucleus. During mitosis, metazoan NPCs disassemble into approximately a dozen subunits. A subset of vertebrate nucleoporins are targeted to mitotic kinetochores. Kinetochores are the primary site of attachment for chromosomes to the microtubules of the mitotic spindle, and thus mediate the correct segregation of genetic material into daughter cells at anaphase.
At least two nucleoporin sub-complexes are targeted to mitotic kinetochores. First, we previously reported that the RanBP2 complex associates with kinetochores in a microtubule-dependent manner. The complex consists of RanBP2, SUMO-1-conjugated RanGAP1 (the activating protein for the Ran GTPase), and Ubc9 (the sole conjugating enzyme for the SUMO family of ubiquitin-like modifiers). Second, our recent studies focused on the nine-protein vertebrate Nup107-160 complex, which includes Nup160, Nup133, Nup107, Nup96, Nup85, Nup43, Nup37, Sec13, and Seh1. The Nup107-160 complex is broadly distributed on spindles during prometaphase. It remains kinetochore-bound throughout mitosis and shows enhanced accumulation on unattached kinetochores. Earlier findings demonstrated that Nup107-160 promotes spindle assembly in a context that is distinct from its role in interphase NPCs. During telophase, Nup107-160 is targeted to chromosomes and acts in a critical and early fashion during NPC re-assembly. We have documented a role for this complex in the formation of kinetochore fibers (k-fibers), the microtubule bundles connecting kinetochores to spindle poles.
Unattached kinetochores nucleate microtubules in a manner that is regulated by the Ran GTPase; such microtubules contribute to k-fiber assembly. We found that Nup107-160 interacts with the gamma-tubulin ring complex (gamma-TuRC), an essential and conserved microtubule nucleator, and recruits gamma-TuRC to unattached kinetochores. Our data indicate that Nup107-160 and gamma-TuRC act cooperatively to promote spindle assembly through microtubule nucleation at kinetochores: HeLa cells lacking Nup107-160 or gamma-TuRC were profoundly deficient in kinetochore-associated microtubule nucleation. Moreover, co-precipitated Nup107-160/gamma-TuRC complexes nucleated microtubule formation in assays using purified tubulin. Although Ran did not regulate microtubule nucleation by gamma-TuRC alone, Nup107-160/gamma-TuRC complexes required Ran-GTP for microtubule nucleation. Collectively, our observations show that Nup107-160 promotes spindle assembly through Ran-GTP–regulated nucleation of microtubules by gamma-TuRC at kinetochores and perhaps other sites within mitotic spindles. These observations suggest an important and novel relationship between the NPC and the microtubule cytoskeleton.
Our future studies will focus on a number of issues, including the component(s) at kinetochores that is directly involved in Crm1 recruitment, the relationship between the Nup107-160 and RanBP2 complexes during mitosis, and how they together regulate the attachment of microtubules to kinetochores and the spindle assembly checkpoint. We are also examining the mitotic localization and function of other NPC components.
Additional Funding
- Pharmacology Research and Training Award (PRAT Fellowship) to K.L. 10/2009-9/2011.
Publications
- Mukhopadhyay D, Arnaoutov A, Dasso M. The SUMO protease SENP6 iseEssential for inner kinetochore assembly. J Cell Biol. 2010;188:681-692.
- Kolli N, Mikolajczyk J, Drag M, Mukhopadhyay D, Moffitt N, Dasso M, Salvesen G, Wilkinson KD. Distribution and paralog specificity of mammalian deSUMOylating enzymes. Biochem J. 2010;430:335-344.
- Ryu HJ, Al-Ani G, Deckert K, Kirkpatrick D, Gygi S, Dasso M, Azuma Y. PIASy mediates SUMO-2/3 conjugation of poly (ADP-ribose) polymerase 1 (PARP1) on mitotic chromosomes in vertebrates. J Biol Chem. 2010;285:14415-14423.
- Mukhopadhyay D, Dasso M. The fate of metaphase kinetochores is weighed in the balance of SUMOylation during S phase. Cell Cycle. 2010;9:3194-3201.
- Mishra RK, Chakraborty P, Arnaoutov A, Fontoura BMA, Dasso M. The Nup107-160 complex and γ-TuRC regulate microtubule polymerization at kinetochores. Nat Cell Biol. 2010;12:164-1699.
- Wang Y, Mukhopadhyay D, Mathew S, Hasebe T, Azuma Y, Kolli Y, Shi YB, Wilkinson KD, Dasso M. Identification and developmental expression of Xenopus laevis SUMO proteases. PLoS One. 2009;4:e8462.
- Wang Y, Dasso M. SUMOylation and deSUMOylation at a glance. J Cell Sci. 2009;122:4249-4252.
- Chakraborty P, Seemann J, Mishra RK, Wei J-H, Levay A, Nussenzveig DR, Heiber J, Barber GN, Dasso M, Fontoura BMA. VSV induces spindle damage and nuclear formation defects via matrix protein. EMBO Rep. 2009;10:1154-1160.
- Samoshkin A, Arnaoutov A, Jansen LET, Ouispenski I, Dye L, Karpova T, McNally J, Dasso M, Cleveland DW, Strunnikov A. Human condensin function is essential for centromeric chromatin assembly and proper sister kinetochore orientation. PLoS One. 2009;4:e6831.
Collaborators
- Beatriz M. Fontoura, PhD, University of Texas Southwestern Medical Center, Dallas, TX
- Alexander Strunnikov, PhD, Program on Cellular Regulation and Metabolism, NICHD, Bethesda, MD
- Keith D. Wilkinson, PhD, Emory University, Atlanta, GA
- Alfred Yergey, PhD, Biomedical Mass Spectrometry Facility, NICHD, Bethesda, MD
- Yoshiaki Azuma, PhD, University of Kansas, Lawrence, KS
Contact
For more information, email mdasso@helix.nih.gov or visit sccr.nichd.nih.gov.