You are here: Home > Section on Protein Biosynthesis
Mechanism and Regulation of Eukaryotic Protein Synthesis
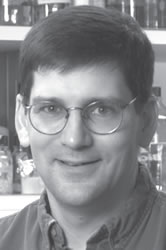
- Thomas E. Dever, PhD, Head, Section on Protein Biosynthesis
- Cheryl G. Bolinger, PhD, Postdoctoral Fellow
- Chune Cao, Biological Laboratory Technician
- Joo-Ran Kim, BS, Special Volunteer
- Yvette R. Pittman, PhD, Postdoctoral Fellow
- Stefan Rothenberg, PhD, Visiting Fellow
- Preeti Saini, PhD, Visiting Fellow
- Byung-Sik Shin, PhD, Staff Scientist
- Meghna Thakur, PhD, Visiting Fellow
We study the mechanism and regulation of protein synthesis, focusing on GTPases and protein kinases that control this fundamental cellular process. We use molecular-genetic and biochemical studies to dissect the structure-function properties of the translation initiation factors eIF2, a GTPase that binds methionyl-tRNA to the ribosome, and eIF5B, a second GTPase that catalyzes ribosomal subunit joining in the final step of translation initiation. Our studies have revealed a critical role for eIF2 in start codon selection and have defined a functionally important contact between eIF5B and the ribosome. We also investigate stress-responsive protein kinases that phosphorylate eIF2alpha. Recent studies revealed fast evolution of the antiviral kinase PKR in vertebrates and linked this to altered sensitivity to poxvirus inhibitors of the kinase. Finally, our studies on the factor eIF5A revealed an unanticipated role in the elongation phase of protein synthesis.
Structure-function analysis of the universally conserved translational GTPase eIF5B/IF2
We have collected families with CNC and related syndromes from several collaborating institutions worldwide. Through genetic linkage analysis, we identified loci harboring genes for CNC on chromosomes 2 (2p16) and 17 (17q22–24) and are currently searching for other possible loci for this genetically heterogeneous condition. With the application of state-of-the-art molecular cytogenetic techniques, we are investigating the participation of these currently identified genomic loci in the expression of the disease and have constructed a comprehensive genetic and physical map of the 2p16 chromosomal region for cloning the CNC-associated sequences from this region. Studies in cultured primary tumor cell lines (established from our patients) identified a region of genomic amplification in CNC tumors in the center of the map. The PRKAR1A gene on 17q22–24, which is the gene responsible for CNC in most cases of the disease, appears to undergo loss of heterozygosity in at least some CNC tumors. PRKAR1A is also the main regulatory subunit (subunit type 1-α) of PKA, a central signaling pathway for many cellular functions and hormonal responses. We have increased the number of CNC patients in genotype-phenotype correlation studies, which are expected to provide insight into the complex biochemical and molecular pathways regulated by PRKAR1A and PKA. We expect to identify new genes by ongoing genome-wide searches for patients and families who do not carry PRKAR1A mutations.
In the final step of translation initiation, the large 60S ribosomal subunit joins with the 40S subunit, already bound to an mRNA, to form an 80S ribosome that is competent for protein synthesis. We previously discovered the translation initiation factor eIF5B, an ortholog of the bacterial translation factor IF2, and showed that it catalyzes ribosomal subunit joining. The eIF5B is a GTPase that binds to GTP and hydrolyzes the nucleotide in the presence of 80S ribosomes. Moreover, we showed that GTP hydrolysis by eIF5B is a regulatory switch governing the release of eIF5B from the ribosome following subunit joining. Our current efforts aim to elucidate eIF5B's structure-function properties and to understand the role played by eIF5B in GTP binding and hydrolysis.
The function of both eIF5B and the translational GTPases that promote elongation and termination of protein synthesis relies on proper interaction with the ribosome. While structural studies using purified proteins have revealed binding sites for the GTPases on the ribosome, in vivo data supporting these sites have not been reported. Using molecular-genetic studies in the yeast Saccharomyces cerevisiae, we showed that, due to failure of eIF5B to dissociate from the ribosome following subunit joining, mutations that impair GTP hydrolysis by eIF5B impair yeast cell growth. In suppressor screens, we identified intragenic mutations in domain II of eIF5B that suppress the toxic effects associated with expression of the GTPase-deficient eIF5B-H480I mutant in yeast. Moreover, we identified a mutation in helix h5 of the 18S rRNA in the 40S ribosomal subunit that likewise suppresses the growth defects of yeast expressing the eIF5B-H480I mutant. Both the rRNA and intragenic mutations lowered the ribosome binding affinity of eIF5B, indicating that the mutations enable eIF5B release from the ribosome in the absence of GTP hydrolysis. Linking the hydroxyl radical generator BABE at the sites of the domain II suppressors in eIF5B, we mapped the region of the ribosome contacted by domain II of eIF5B. Interestingly, the domain II suppressors contacted the body of the 40S subunit in the vicinity of helix h5. Thus, the rRNA and domain II suppressors affect the same contact surface between eIF5B and the 40S ribosomal subunit. Given that the helix h5 mutation also impairs translation elongation factor function, we propose that the rRNA and eIF5B suppressor mutations provide in vivo evidence supporting a functionally important docking of domain II of the translational GTPases on the body of the small ribosomal subunit (see reference 1).
The eIF5B resembles a chalice, with the a-helix H12 forming the stem connecting the GTP-binding domain cup to the domain IV base. Helix H12 has been proposed to function as a rigid lever arm governing domain IV movements in response to nucleotide binding and as a molecular ruler fixing the distance between domain IV and the G domain of the factor. In an ongoing study to investigate its function, we altered the length and rigidity of helix H12. While helix H12 mutations had minimal impacts on GTP binding and on eIF5B ribosome binding and GTPase activities, shortening the helix impaired the rate of subunit joining in vitro. Moreover, both shortening the helix and increasing its flexibility impaired the stability of Met-tRNA bound to the 80S product of subunit joining. These data support the notion that helix H12 functions as a ruler connecting the GTPase center of the ribosome to the P site where Met-tRNA is bound and that helix H12 rigidity is required to stabilize Met-tRNA binding.
Molecular analysis of eIF2alpha protein kinase activation, substrate recognition, and evolution
Phosphorylation of eIF2alpha is a common mechanism for downregulating protein synthesis under stress conditions. Four distinct kinases phosphorylate eIF2alpha on Ser51 under different cellular stress conditions. GCN2 responds to amino acid limitation, HRI to heme deprivation, PERK to ER stress, and PKR to viral infection. Consistent with their common activity to phosphorylate eIF2alpha on Ser51, the kinases show strong sequence similarity in their kinase domains. Phosphorylation of eIF2alpha converts eIF2 from a substrate to an inhibitor of its guanine-nucleotide exchange factor eIF2B. The inhibition of eIF2B impairs general translation, thereby slowing the growth of yeast cells and, paradoxically, enhancing the translation of the GCN4 mRNA required for yeast cells to grow under amino-acid starvation conditions. We used structural, molecular, and biochemical studies to define how the eIF2alpha kinases recognize their substrate.
In collaboration with Frank Sicheri, we obtained the X-ray structure of eIF2alpha bound to the catalytic domain of PKR. Back-to-back dimerization enables each PKR protomer to engage a molecule of eIF2alpha in the crystal structure. Given that all four eIF2alpha kinases share the PKR residues mediating kinase domain dimerization and eIF2alpha recognition, we propose that all four kinases similarly dimerize and recognize eIF2alpha. Based on our results, we propose an ordered mechanism of PKR activation by which catalytic domain dimerization triggers autophosphorylation on Thr446, a reaction that in turn is required for specific eIF2alpha substrate recognition.
Our previous molecular-genetic studies of PKR were useful for uncovering how dimerization of PKR serves to regulate catalytic activation; therefore, in ongoing studies we employed a similar strategy to gain insight into how PKR gains access to the Ser51 phosphorylation site in eIF2alpha. In the crystal structure of the PKR–eIF2alpha complex, the C-terminal lobe of the kinase contacts eIF2alpha on a face remote from Ser51, leaving Ser51 about 20 Å from the kinase active site. PKR mutations, such as PKR-T487A, that cripple the eIF2alpha-binding site impair phosphorylation, and we identified mutations in eIF2alpha that suppress the PKR-T487A mutation, increase Ser51 loop mobility, and restore phosphorylation both in vivo and in vitro. These eIF2alpha mutations either disrupt a hydrophobic network that restricts the position of Ser51 or alter a linkage between the PKR-docking region and the Ser51 loop. Finally, we found that addition of PKR increases the protease sensitivity of the Ser51 loop in eIF2alpha. We propose that restricted mobility of Ser51 in free eIF2alpha prevents promiscuous phosphorylation and the attendant translational regulation by heterologous kinases, whereas binding of PKR to eIF2alpha triggers a signal that induces a conformational change of the phosphorylation site sequences and enables Ser51 to access the PKR active site.
Phylogenetic analyses of the eIF2alpha kinases as well as four unrelated protein kinases revealed fast evolution of the PKR kinase domain in vertebrates. These evolutionary studies also revealed evidence of positive diversifying selection at specific sites in the PKR kinase domain. Substitution of positively selected residues in human PKR with residues found in other species altered the sensitivity to PKR inhibitors from different poxviruses (see reference 2). Moreover, a comparison of the sensitivity of human and mouse PKR to poxviral pseudosubstrate inhibitors revealed differences that were traced to positively selected residues near the eIF2alpha binding site. Our results indicate how an antiviral protein (PKR) evolved to evade viral inhibition while maintaining its primary function (phosphorylation of eIF2alpha). Moreover, our identification of species-specific differences in PKR susceptibility to viral inhibitors has important implications for studying human infections in nonhuman model systems.
Poxvirus regulation of protein kinase PKR
As part of the mammalian cell's innate immune response, the double-stranded RNA–activated protein kinase PKR phosphorylates the translation initiation factor eIF2α to inhibit protein synthesis and thus block viral replication. To subvert this host cell defense mechanism, viruses produce inhibitors of PKR. Several members of the poxvirus family express two types of PKR inhibitor: a pseudosubstrate inhibitor and a double-stranded RNA–binding protein called E3L. The vaccinia virus K3L protein resembles the N-terminal third of eIF2alpha, with both proteins containing a beta-barrel fold of the OB-fold family. Whereas high-level expression of human PKR was toxic in yeast, co-expression of the vaccinia virus K3L protein or the related variola (smallpox) virus C3L protein abrogated such growth inhibition. We used this yeast assay to identify PKR mutants resistant to K3L inhibition. Twelve mutations were identified and found to map to the C-terminal lobe of the PKR kinase domain in the vicinity of the eIF2alpha binding site. The PKR mutations specifically conferred resistance to the K3L protein, but not to the E3L protein, both in yeast and in vitro. Biochemical studies revealed that the mutations did not affect eIF2alpha phosphorylation, but led to a nearly 15-fold decrease in K3L binding affinity. Our results indicate that subtle changes in the PKR kinase domain can drastically affect pseudosubstrate inhibition while leaving substrate phosphorylation intact. We propose that these paradoxical effects of the PKR mutations on pseudosubstrate versus substrate interactions reflect differences between the rigid K3L protein and the plastic nature of eIF2alpha around the Ser51 phosphorylation site (see reference 3), as exemplified in our ongoing studies revealing the importance of an induced conformational change to expose Ser51 for phosphorylation.
In related studies, we are identifying and characterizing PKR mutants resistant to inhibition by the E3L protein. These PKR mutations map to both the kinase domain dimerization interface and the N-terminal regulatory (double-stranded RNA–[dsRNA] binding) domain of the protein. Consistent with the distinct nature of the two poxvirus inhibitors of PKR, the PKR mutations that confer resistance to E3L do not confer resistance to K3L. The identification of E3L-resistant mutations in the PKR dimerization interface is consistent with the notion that E3L inhibits PKR by forming inactive heterodimers with the kinase.
Molecular analysis of the hypusine-containing protein eIF5A
The translation factor eIF5A, the sole protein containing the unusual amino acid hypusine [Ne-(4-amino-2-hydroxybutyl)lysine], was originally identified based on its ability to stimulate the yield (endpoint) of methionyl-puromycin synthesis, a model assay for first peptide bond synthesis. However, the precise cellular role of eIF5A is unknown. Using molecular-genetic and biochemical studies, we recently showed that eIF5A promotes translation elongation and that this activity is dependent on the hypusine modification. As eIF5A is a structural homolog of the bacterial protein EF-P, we propose that eIF5A/EF-P is a universally conserved translation elongation factor (see reference 4).
Additional Funding
- Trans-NIH/FDA Intramural Biodefense Research Program Award (2009-2010, ongoing)
Publications
- Shin B-S, Kim J-R, Acker MG, Maher KN, Lorsch JR, Dever TE.. rRNA suppressor of a eukaryotic translation initiation factor 5B/initiation factor 2 mutant reveals a binding site for translational GTPases on the small ribosomal subunit. Mol Cell Biol. 2009; 29:808-821.
- Rothenburg S, Seo EJ, Gibbs JS, Dever TE, Dittmar K. Rapid evolution of protein kinase PKR alters sensitivity to viral inhibitors. Nat Struct Mol Biol. 2009; 16:63-70.
- Seo EJ, Liu F, Kawagishi-Kobayashi M, Ung TL, Cao C, Dar AC, Sicheri F, Dever TE. Protein kinase PKR mutants resistant to the poxvirus pseudosubstrate K3L protein. Proc Natl Acad Sci U S A 2008; 105:16894-16899.
- Saini P, Eyler DE, Green R, Dever TE. Hypusine-containing protein eIF5A promotes translation elongation. Nature 2009; 459:118-121.
- Lorsch JR, Dever TE. Molecular view of 43S complex formation and start site selection in eukaryotic translation initiation. J Biol Chem. 2010; 285:21203-21207.
Collaborators
- Michael G. Acker, PhD, The Johns Hopkins University, Baltimore, MD
- Katharina Dittmar, PhD, SUNY-Buffalo, Buffalo, NY
- Daniel E. Eyler, PhD, The Johns Hopkins University, Baltimore, MD
- James S. Gibbs, PhD, Laboratory of Viral Diseases, NIAID, NIH, Bethesda, MD
- Rachel Green, PhD, The Johns Hopkins University, Baltimore, MD
- Jon R. Lorsch, PhD, The Johns Hopkins University, Baltimore, MD
- Frank Sicheri, PhD, Samuel Lunenfeld Research Institute, Mount Sinai Hospital, and University of Toronto, Toronto, Canada
Contact
For more information, email tdever@box-t.nih.gov or visit spb.nichd.nih.gov