You are here: Home > Unit on Cellular Communication
Biochemical Genetics of Signal Transduction
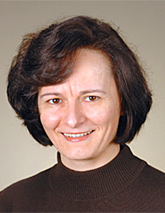
- Mihaela Serpe, PhD, Head, Unit on Cellular Communication
- Young-Jun Kim, PhD, Visiting Fellow
- Peter Nguyen, Biological Laboratory Technician
- Carolyn E. Peluso, PhD, Postdoctoral Fellow
We study molecular mechanisms that regulate cell-cell communication during development. In all multicellular organisms, the identity, movements, and ultimate function of cells must be coordinated with those of their neighbors. These processes are largely guided by highly conserved signaling molecules, which provide spatial and temporal information to the developing tissues. Deciphering the molecular nature of mechanisms that guide development and confer robust developmental patterning can be aided by the fact that successful strategies are repeated throughout evolution, although selective pressures tune the properties of individual components.
We focus on signaling by TGF-beta of growth and differentiation factors, one of the largest families of signaling molecules, highly conserved throughout the animal kingdom. TGF-beta signaling factors have the ability to function as morphogens, that is, to specify cell fate in a concentration-dependent manner. Also, these signaling factors provide a mechanism for coupling a cell to its environment, so that the cell has the necessary plasticity to respond appropriately to changes in its environment or even to its own state. We use the Drosophila melanogaster model system and a variety of molecular and biochemical approaches to study genes that modulate the function of TGF-beta–type signals. We are interested in how extracellular availability of the signaling factors is regulated in time and space and in the role of the cell surface in modulating the local activation of TGF-beta-type signaling.
Shaping BMP morphogen gradients through enzyme-substrate interactions
Spatially non-uniform distributions of secreted morphogens guide tissue development in a highly reproducible and robust manner. In early embryonic patterning of insects and chordates, BMPs guide dorsal/ventral patterning through a complex network that relies on the spatial distribution of a BMP ligand (Dpp, BMP2/4) and a BMP antagonist (Sog/Chordin). The regulation of Sog in Drosophila and Chordin in vertebrates has, however, acquired distinct characteristics. We use biochemical, genetic, and computational modeling studies to explore the molecular etiology of these differences.
In the early Drosophila embryo, laterally secreted Sog binds to Dpp in a complex that inhibits Dpp signaling locally and facilitates long-range ligand diffusion, shuttling Dpp from the lateral domain towards the dorsal midline. Sog plays both positive and negative roles in regulating BMP activity, a phenomenon previously referred to as the "Sog paradox." The negative role comes from blocking access of ligands to receptors; the positive effect comes from Sog's ability to facilitate Dpp diffusion. A key component that helps create flux is the processing of Sog by Tolloid (Tld), a metalloprotease of the BMP-1 family. The net movement of Dpp dorsally is generated by reiterated cycles of complex formation, diffusion, and destruction by Tld. Without Tld, too much Sog traps the BMP-type ligand, and the dorsal structures fail to form. Without Sog there is no net movement of Dpp dorsally, the peak signaling domain does not form, amnioserosa—the tissue that requires peak BMP signaling—is not specified, and the embryos fail to develop and die.
Although Chordin is thought to be the functional homolog of Sog, when introduced into Drosophila , it only acts as an inhibitor and cannot promote long-range Dpp signaling. One biochemical difference between these two molecules is that processing of Sog by Tld requires the BMP ligand as an obligatory co-substrate, while Chordin does not. We propose that Sog's ability to function as the more efficient BMP transporter—that results in long-range BMP signaling—resides, in molecular terms, in the co-substrate requirements for Tld-mediated Sog degradation. This predicts that a "Chordin-like" Sog, which is independent of BMP binding for its destruction, would resemble Chordin when introduced in fly embryos and be less efficient in shuttling of BMP-type ligands.
To test this hypothesis, we first characterized, on a molecular level, the Tld processing sites in Sog and compared them with processing sites in Chordin. We derived a consensus cleavage recognition sequence for the Tld processing sites in Sog. We used this peptide to study the enzyme-substrate interactions in Sog and compared them with Chordin sequences. From this modeling, we hypothesized that several residues at the processing site might be responsible for making one substrate, but not the other, dependent on BMP binding for processing. Despite the fact that Sog and Chordin are only 22% identical, we indeed found that only a limited number of residues surrounding the processing site were responsible for making Sog processing dependent on BMP binding, while Chordin is not. We generated Sog variants that are BMP-independent Tld substrates in vitro (Sog-i variants) and found that Sog-wt and Sog-i were otherwise indistinguishable in their binding to BMP ligands and/or ability to inhibit their signaling.
To test the biological effect of the Sog variants on the BMP morphogen gradient profile, we constructed transgenic fly lines that permitted normal spatial and temporal expression of Sog proteins at endogenous levels. Replacement of endogenous Sog with "Chordin-like" variants changed the steep BMP gradient found in Drosophila embryos to a shallower profile, one more characteristic of that observed in some vertebrate embryos. This change in the BMP profile had a major impact on Drosophila dorsal patterning: first, it affected cell allocation and consequently the size of the ensuing tissue; secondly, it resulted in increased variability of patterning across individual populations.The biological consequences of replacing Sog with Sog-i could not be explained simply by quantity differences between the sog-wt and sog-itransgenes. First, we observed shallow BMP gradient profiles and increased cell allocation/amnioserosa fields using multiple independent sog-itransgenic lines with expression levels comparable with those of the sog-wt lines. Secondly, additional copies of sog-wt and sog-itransgenes did not significantly impact the BMP gradient profiles or amnioserosa fields. This suggests a more profound change in Sog-i—likely in its ability to facilitate diffusion of BMP complexes towards the dorsal midline. To further search for alternative explanations for the Sog-i effects, we collaborated with David Umulis's group to modify a 3D embryonic computational model to include variants that differ in Sog's biophysical parameters. Indeed, modeling suggests that the co-substrate requirement for Sog processing by Tld is critical for proper Dpp gradient formation. In computations that relax this constraint and allow for Sog degradation when not complexed with Dpp, Dpp flux towards the dorsal midline is greatly reduced. Furthermore, our experimental observations could be not captured by any changes in Sog affinity to Dpp; instead, a modest increase in the processing of Sog by Tld without Dpp resulted in signaling profiles that matched the experimental data very well.
Our results also provide an intriguing alternative explanation to an open debate in the field: BMP-dependent Sog processing does not confer robustness against fluctuations in sog copy number, but it does provide more consistent, reliable patterning between individuals within a population of the same genotype. Thus, BMP-dependent Sog destruction appears to reduce embryo-to-embryo variability between individuals in a population and provide robust patterning of the dorsal structures.
Taken together, our results indicate that a "Chordin-like" Sog cannot reliably support patterning of the early Drosophila embryo. By modifying the Sog-Tld substrate-enzyme interaction with just a few residue changes, a new developmental function for Sog evolved that ensured reliable shuttling of BMPs and robust patterning.
Characterization of molecular mechanisms that spatially restrict Tolloid activities
The BMP-1/Tolloid proteases are developmentally important enzymes that must be very tightly regulated. Drosophila has two highly homologous enzymes of this family: Tld and Tolloid-related (Tlr). During early embryo patterning, the secreted Tld acts cell-autonomously. Tlr acts later in development and circulates in the hemolymph, posing the risk of promiscuous proteolysis when inappropriately active. We proposed that cell tethering and cell-surface catalysis constitute an important level of regulation for Tld-type proteins, and we have initiated a project to identify and characterize component(s) that act to localize Tlds activities.
In mammals, one function of BMP-1/Tld enzymes is to process the inhibitory pro-peptides of certain TGF-beta–type ligands such as myostatin and GDF11, which activates the ligand in the circulatory system. In Drosophila , we found that Tlds can cleave and destabilize the pro-domains of several TGF-beta ligands including activin (Act), the activin-like protein dawdle (Daw), and myoglianin (Myo). In the case of Daw, pro-peptide cleavage enhances the signaling activity of the ligand, and this is required for normal guidance of motor axons en route to their muscle targets. In the absence of Tlr, Daw, or any other activin pathway components, the motor neurons fail to defasciculate from their nerve tracks. Previous work suggested that the Daw signaling pathway is activated in motor neurons by ligand secreted from muscles and/or glial cells. An important question is to determine if and how this pathway is locally activated by Tlr, a circulatory enzyme.
To address this problem, we generated membrane-tethered Tlr variants that remained associated with the extracellular membranes both in vitro and in vivo. Using these reagents, we have begun to test the spatial and temporal requirements for the Tlr enzymatic activity. We have found that Tlr does not circulate in an inactive form, i.e., pro-enzyme, in the hemolymph of Drosophila larvae; instead, Tlr circulates as an active enzyme, with the inhibitory pro-peptide already removed from it. Secondly, when various tlr transgenes were ubiquitously expressed in tlr null mutants, we found that the levels of circulatory Tlr (as measured by Western blots in larval hemolymph samples) directly correlated with the penetrance of axon guidance defects in larvae, as well as with the adult viability. Relatively high levels of circulatory, active Tlr were required to fully rescue the phenotypical defects and the viability. Thus, the fly hemolymph appeared to accommodate significant amounts of active Tlr enzyme. Lastly, the axon guidance defects of tlr null mutants were mildly reduced by expressing a membrane-tethered Tlr protein in the muscles; for example, the penetrance of defects at the 12/13 muscle cleft decreased from 47% in tlr mutants to 38% in partially rescued animals. In contrast, when the membrane-tethered Tlr was provided in neurons, the mis-innervation rates dropped to wild-type levels, and the resulted animals appeared fully rescued, suggesting that the Tlr activity must be concentrated at the surface of neurons.
Future studies will focus on characterization of cell-specific component(s) and mechanisms that help localize Tlr activity at the surface of the motor neurons.
Collaborators
- Seth S. Blair, PhD, University of Wisconsin, Madison, WI
- Chi-Hon Lee, PhD, Program on Cellular Regulation and Metabolism, NICHD, Bethesda, MD
- Michael B. O’Connor, PhD, University of Minnesota, Minneapolis, MN
- David Umulis, PhD, Purdue University, West Lafayette, IN
Contact
For more information, email serpemih@mail.nih.gov or visit ucc.nichd.nih.gov.