You are here: Home > Section on Cellular and Membrane Biophysics
Membrane Remodeling During Viral Infection, Parasite Invasion, and Apoptosis; Components and Kinetics in Exocytosis
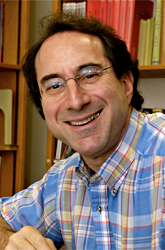
- Joshua Zimmerberg, MD, PhD, Head, Section on Cellular and Membrane Biophysics
- Pavel Bashkirov, MS, Guest Researcher
- Ludmila Bezrukov, MS, Contractor
- Paul S. Blank, PhD, Staff Scientist
- Alexander Chanturiya, PhD, Guest Researcher
- Jane E. Farrington, MS, Guest Researcher
- Vadim A. Frolov, PhD, Senior Research Fellow
- Svetlana Glushakova, PhD, Staff Scientist
- Glen Humphrey, PhD, Guest Researcher
- Vladimir A. Lizunov, MS, Visiting Fellow
- Julia Mazar, PhD, Visiting Fellow
- Gulcin Pekkurnaz, MS, Postdoctoral Fellow
- Anna Shnyrova, MS, Predoctoral Fellow
We study membrane mechanics, intracellular molecules, membranes, viruses, organelles, and cells in order to understand viral and parasite infection, exocytosis, apoptosis, the mechanism of immune protection by stem cells and their cytotoxic potential, and immune dysfunction in microgravity. The overall goals of the exocytosis projects are to understand the mechanisms of cellular secretion at the physical, biophysical, and chemical levels. This process of protein secretion is the climax of the secretory pathway, and it operates in both constitutive and triggered ways. The process of endocytosis is equally important to retrieve membrane components.
This year, we focused on the regulation of insulin-induced control of glucose transport into fat cells. We found that insulin controls the spatial distribution of the glucose transporter GLUT4 on the cell surface by regulating its post-fusion dispersal. While GLUT4 is fundamental to insulin-regulated glucose metabolism, its dynamic spatial organization in the plasma membrane (PM) is unclear. We demonstrated that insulin regulates not only the exocytosis of GLUT4 storage vesicles, but also PM distribution of GLUT4 itself. In the basal state, domains (clusters) of GLUT4 molecules in the PM are created by an exocytosis that retains GLUT4 at the fusion site. Surprisingly, when insulin induces a burst of GLUT4 exocytosis, it does not merely accelerate this basal exocytosis, but rather stimulates 60-fold another mode of exocytosis that disperses GLUT4 into the PM. By contrast, internalization of most GLUT4, regardless of insulin, occurs from pre-existing clusters via the subsequent recruitment of clathrin. The data fit a new kinetic model that features multifunctional clusters as intermediates of exocytosis and endocytosis. Thus, GLUT4 exists in the PM as freely-diffusing molecules and stable clusters, clusters/domains are generated by fusion with selective retention of GLUT4, GLUT4 is internalized at the clusters via subsequent recruitment of clathrin, and insulin induces a burst of GLUT4 exocytosis that disperses GLUT4 directly into the PM.
The overall goal of the remodelling project is to determine the physico-chemical mechanisms of membrane remodelling in cells. These processes underlie many pathological processes, such as E. coli toxicosis, malarial infection, viral infection, and the failure of apoptosis in cancer. This year we report specifically on two basic projects: 1) elucidating the egress pathway of the malarial parasite Plasmodium falciparum in normal red blood cells and those of patients with sickle cell anemia, and 2) reconstructing a human erythrocyte infected with P. falciparum in three dimensions using a novel modality of electron microscopy. In the first project, we studied the, as yet unknown, mechanism of parasite egress from erythrocytes infected with P. falciparum, the apicomplexan parasite that causes malignant malaria. How such egress disseminates parasites in the host at the end of each asexual cycle is unknown. We found two new stages of the egress program: (a) swelling of the parasitophorous vacuole accompanied by shrinkage of the erythrocyte compartment; and (b) poration of the host cell membrane seconds before erythrocyte rupture because of egress. Egress was inhibited in dehydrated cells from patients with sickle cell disease as happens with experimental dehydration of normal cells, suggesting that vacuole swelling involves intake of water from the erythrocyte compartment. Erythrocyte membrane poration occurs in relaxed cells, thus excluding involvement of osmotic pressure in this process. We suggest the following egress program: parasites initiate water influx into the vacuole from the erythrocyte cytosol to expand the vacuole for parasite separation and vacuole rupture upon its critical swelling. Separated parasites leave the erythrocyte by breaching its membrane, weakened by putative digestion of erythrocyte cytoskeleton and membrane poration.
Electron tomography provides three-dimensional structural information about supramolecular assemblies and organelles in a cellular context, but image degradation, caused by scattering of transmitted electrons, limits applicability in specimens thicker than 300 nm. In the second project, we showed that scanning transmission electron tomography of 1000 nm thick samples using axial detection provides resolution comparable to conventional electron tomography. We demonstrated the method by reconstructing a human erythrocyte infected with P. falciparum.
Insulin regulates fusion of GLUT4 vesicles independent of Exo70-mediated tethering
Insulin regulates glucose transport through recruitment of GLUT4 to the PM where these transporters facilitate glucose uptake. The current model of GLUT4 recycling proposes a complex regulated system of GLUT4 cycling among specialized GLUT4 storage vesicles (GSV), intracellular compartments, and the PM. Insulin has been variably reported to regulate GLUT4 recycling in several ways. However, despite the large number of processes apparently affected by insulin, recent experimental work by us and others strongly suggests that the main site of regulation of GLUT4 recycling and glucose uptake occurs at the PM.
To probe both GLUT4 organization in the PM and its relationship to insulin-regulated recycling, we investigated GLUT4 dynamics in isolated rat adipose cells. We find: 1) clusters are generated by fusion with retention of GLUT4 in nascent domains; 2) GLUT4 is internalized at the domains after subsequent recruitment of clathrin, and 3) insulin induces a burst of GLUT4 exocytosis that mostly bypasses the domains and disperses GLUT4 directly into the PM.
The relationship between the spatial and temporal organization of PM glucose transporters is key to the regulation of cell metabolism. In addition to the complex recycling of GLUT4 among endosomal compartments, GLUT4-storage vesicles (GSV), and the PM, we now know that their spatial organization in the PM, where they facilitate glucose transport, depends upon insulin in a time-dependent fashion. The predominance in the basal state of PM GLUT4 cluster formation, upon exocytosis of GSV, gives way with insulin stimulation to a shift in GSV fusion to GLUT4 dispersal in the PM. Further, GLUT4 clusters are found to nucleate clathrin assembly, where most of the GLUT4 internalization from the cell surface then takes place. Thus, GLUT4 clusters represent a molecular organization that mediates the transition between GLUT4 delivery and withdrawal from the PM, depending upon insulin.
While no molecular mechanism for GLUT4 clustering has been established, it was often attributed to accumulation of GLUT4 either in clathrin-coated pits or caveolae. It has been proposed that caveolar structures play some intermediate role in GLUT4 internalization. In our experiments we did not detect any significant co-localization of GLUT4 and caveolin in either basal or insulin-stimulated cells, which is consistent with another recent study arguing against a direct role of caveolae in GLUT4 recycling.
Though the role of clathrin in the recycling of GLUT4 is well supported, there is no evidence to suggest direct involvement of clathrin in GLUT4 clustering. We were able to separately measure the overall co-localization of GLUT4 and clathrin, as well as co-localization of surface-exposed GLUT4 with clathrin. To our surprise, we found that surface-exposed GLUT4 is far less co-localized with clathrin than total GLUT4. Thus, a specific accumulation of GLUT4 in clathrin-coated pits is unlikely; it is more likely that these two molecules co-localize in intracellular compartments. Together with the fact that the majority of GLUT4 clusters exists at the PM without showing any co-localization with clathrin, our data indicate that clathrin itself cannot account for the existence and formation of GLUT4 clusters at the PM.
The delivery of GLUT4 to the PM through insulin-regulated exocytosis has been well documented by both biochemistry and live-cell imaging. Using TIRF microscopy, a number of groups detected single GSV fusion events, using the post-fusion dispersal of fluorescently labeled GLUT4-GFPas a criterion for fusion. However, the number of fusion events measured microscopically was fewer than the number expected from biochemical and physiological approaches. Surprisingly, we found that many fusion events were not associated with dispersal of GLUT4 from the site of fusion, explaining why the number of fusion events detected only by GLUT4 dispersal was underestimated. Fusion-with-retention was predominant in the basal state where we observed that almost all fusion events detected by IRAP-pHluorin flash were not accompanied by dispersal of GLUT4 into the PM. The result of this fusion-with-retention is the creation of de novo GLUT4 clusters at the PM.
Consistent with previous results, insulin increased the overall number of fusion events. Interestingly, insulin not only affected the number of fusion events, but also dramatically shifted the mode of fusion towards fusion with dispersal of GLUT4 into the PM. This finding is also consistent with the pronounced increase of diffuse HA-antibody staining of the PM and corresponding shift in the relative amount of GLUT4 from clusters to monomers, an observation that further implies that, while clustered and monomeric GLUT4 co-exist in a steady state, the relative amounts of GLUT4 in these pools can be differentially regulated by insulin.
Interestingly, the insulin-stimulated increase in GLUT4 fusion was transient, and after a pronounced peak at 2-3 min, the fusion frequency declined to a level only slightly above basal. While old models of GLUT4 recycling predict an overall increase of GLUT4 recycling in response to insulin, our results fit the prediction of a quantum release model. However, while the older model posits that insulin regulates the size of the active pool available for GLUT4 recycling, our model includes all GLUT4 in the recycling process and addresses the existence of GLUT4 clusters as a distinct pool. One important feature of our model, based on the experimental observations, is the restriction of GLUT4 internalization to the clusters. Our data suggest that GLUT4 internalization occurs mainly from clusters via recruitment of clathrin, while other clathrin-coated pits outside the clusters do not efficiently endocytose GLUT4. Such an organization of GLUT4 recycling provides flexibility to upregulate PM GLUT4 (in monomeric states) separately from GLUT4 available for endocytosis (clustered).
Taken together, the data presented in this study suggest that GLUT4 clusters may function as intermediate hubs between the time of GLUT4 exocytosis and their internalization. In the basal state, these domains appear to play the major role in regulating the recycling of GLUT4 between the PM and the intracellular pool of GSV. In the insulin-stimulated state, the rapid increase in PM GLUT4 is achieved by an enhanced GSV fusion, with full and immediate release of GLUT4 molecules diffusely into the PM. However, the rate of internalization and recycling of GLUT4 into GSV must now include a new parameter—the time it takes for GLUT4 to reach an uptake site; the rate-limiting step in this trafficking process remains to be determined. The kinetics, through GLUT4 hubs, must ultimately determine the new equilibrium GLUT4 activity level set by insulin stimulation—of particular interest in pathological states in which the relationship between insulin blood levels and GLUT4 activity is disrupted.
New stages in the program of malaria parasite egress imaged in normal and sickle erythrocytes
Based on amphiphiles, osmotic stress, and protease inhibitors, we hypothesize that egress is pressure-driven through folding and fragmentation of an enzymatically altered erythrocyte membrane. Osmotic pressure could build up in either the parasitophorous vacuole (PV) or the host cell cytoplasm. We propose and test the idea that parasites easily pass through a hemoglobin-depleted erythrocyte cytoplasm and breach a weakened erythrocyte membrane.
The vacuole swells several minutes before parasite egress. Beginning adjacent to the parasite's space, vacuole swelling later extends in all directions. At the same time, the visible area of the erythrocyte compartment shrinks, suggesting redistribution of water between the erythrocyte cytosol and vacuole. Eventually, dissociated parasites leave the host cell by breaching first the PV membrane (PVM)—presumably when PV critical volume is reached—and then the erythrocyte membrane. Thus, dependence of egress on osmotic pressure can be described in terms of erythrocyte hydration, which affects the swelling and rupture of the vacuole. To test the relationship between erythrocyte hydration and parasite egress, we used dehydrated erythrocytes from donors homozygous for sickle hemoglobin gene (HbSS versus normal HbAA). Sickle erythrocytes do support P. falciparum replication, but dehydrated sickle cells did not allow normal egress. The reduced erythrocyte volume may contribute to malarial protection in individuals with sickle erythrocytes. Notably, reduced erythrocyte volume is also a characteristic of individuals with thalassemia and iron deficiency. Alternatively, one may speculate that Plasmodium gained an advantage by targeting reticulocytes, the largest circulating erythrocytes in the host. The negative effect of erythrocyte dehydration on parasite egress, which we demonstrated, and on invasion emphasizes the general importance of host cell hydration for the asexual cycle of malaria parasites.
In this project, we found two new essential steps in P. falciparum egress from erythrocytes. First, the parasitophorous vacuole swells as the erythrocyte shrinks, suggesting ion and water redistribution between these two compartments of infected cells. At the end of the cycle, vacuole swelling apparently provides the space for parasite dissociation prior to egress, leading to vacuole rupture. In the midst of erythrocyte shrinkage, the tension of the erythrocyte membrane decreases without loss of integrity. Second, parasite egress requires host-cell membrane poration prior to host-cell membrane rupture. Membrane poration is observed in erythrocytes that are not swollen and thus does not result from critical membrane stretching. Perhaps either release of protein from erythrocytes or an influx of ions into the host cells, or both, is needed for the asexual parasite cycle to complete. Alternatively, host-cell membrane poration could serve to weaken a barrier that parasites must breach to egress. Poration does not depend on cysteine protease activity, because protease inhibition blocks egress but not poration, and poration is required for the parasite cycle because the membrane sealant P1107 interferes with egress.
Similarities in parasite egress mechanisms between two families of the phylum Apicomplexa—Plasmodium and Toxoplasma—are emerging: both types of parasite make pores in host cell membrane and activate host cell calpain prior to egress. Because P. falciparum has multiple experimental limitations, Toxoplasma, a more conventional organism, is emerging as a model for Apicomplexan biology. Regardless of which organism is studied, egress of parasites is a vital step in diseases devastating humanity. Our appreciation of a more complex egress program provides more targets for novel antimalarials, just as it may help to explain the selective advantage that the sickle trait confers upon its carriers.
Nanoscale 3D cellular imaging by axial scanning transmission electron tomography
Electron tomography (ET) provides a three-dimensional (3D) view of cellular ultrastructure at nanoscale spatial resolution, and thus gives unique insight into the supramolecular basis of biological processes. In this project we investigated an alternative approach to electron tomography that yields 3D reconstructions of thicker (1µm) sections at resolutions comparable to conventional ET of thin sections. The ability to perform 3D reconstructions from larger volumes is particularly attractive for studying unicellular eukaryotic microorganisms, some of which are sufficiently small to be contained within just a few serial sections. Our approach is also valuable for reconstructing entire mammalian cells using serial thick-section tomography.
Scanning transmission electron microscopy (STEM) tomography using a tightly focused electron probe can overcome some of the limitations imposed by conventional ET. First, the ability to focus the probe dynamically in STEM permits in-focus imaging of very large specimen areas even at the highest tilt angles. Second, because in STEM there are no image-forming lenses after the specimen, the resolution in images of thick specimens is not degraded by chromatic aberration.
Generating high-resolution STEM tomograms from entire cells that span several micrometers in depth can be accomplished by imaging serial 1–2 μm–thick sections. We demonstrated the feasibility of reconstructing an entire human erythrocyte infected with P. falciparum from only four consecutive 1 μm–thick sections. Tomogram slices of one infected erythrocyte revealed a parasite during the process of schizogany, i.e. multiple nuclear divisions and formation of new parasites. At this stage of the parasite cycle, active morphogenesis multiplies or produces de novo intracellular organelles for up to 32 new parasites within one schizont. The dynamics of morphogenesis is poorly understood because of the laborious procedure of 3D reconstructions from serial thin sectioning of cells of complex architecture. The spatial resolution achievable with the new method allows us to identify, in thick sections, the major organelles of schizont such as nuclei, rhoptries, pigment vacuole, rough endoplasmic reticulum, Golgi complex, apicoplast, and lipid body. Three layers of membranes surrounding the schizont are clearly identifiable: 1) the parasite plasma membrane, caught at the onset of invagination to form new parasites, 2) the membrane of the parasitophorous vacuole and 3) the erythrocyte membrane. Parasite-derived membrane structures such as tubular extensions of the vacuolar membrane, Maurers clefts, and circular clefts are also visible inside erythrocyte cytoplasm. The data demonstrate that a new ultrastructural method is now available to study the complex dynamics of malaria parasite development inside human erythrocyte. Whereas the process of organellogenesis during schizogeny is currently obscured by the amorphous nature of standard ultrastructural views, the significance of the STEM tomography technique lies in the fact that 10 nm resolution is sufficient to discern organelles. The new technique is rapid enough to permit a series of schizonts to be studied and the morphological sequence of events to be established, as was recently achieved for parasite release. Already, new objects are emerging whose identity is unclear and whose identification will require elaboration of labeling techniques for markers.
Thus STEM tomography using axial detection for imaging thick sections of biological specimens at a spatial resolution around 5-10 nm, which is comparable to the spatial resolution of traditional ET from thin sections (typically 3-8 nm) is both feasible and advantageous. The demand for high-resolution, large-volume imaging of biological specimens has been met so far by the large scale application of traditional ET of thin sections. The present study suggests that it will be possible to reconstruct conveniently and efficiently entire mammalian cells through serial thick-section STEM tomography.
Additional Funding
- Jain Foundation
- DOD CNRM Breast Cancer Program
- Intramural AIDS Targeted Antiviral Program (IATAP)
- Center for Neuroscience & Regenerative Medicine (CNRM)
Publications
- Glushakova S, Mazar J, Hohmann-Marriott MF, Hama E, Zimmerberg J. Irreversible effect of cysteine protease inhibitors on the release of malaria parasites from infected erythrocytes. Cell Microbiol. 2009;11:95-105.
- Hohmann-Marriott MF, Sousa AA, Azari AA, Glushakova S, Zhang G, Zimmerberg J, Leapman RD. Nanoscale 3D cellular imaging by axial scanning transmission electron tomography. Nat Meth. 2009;6:729-731.
- Collins RN, Zimmerberg J. Cell biology: a score for membrane fusion. Nature. 2009;459:1065-1066.
- Mazar J, Thomas M, Bezrukov L, Chanturia A, Pekkurnaz G, Yin S, Kuznetsov SA, Robey PG, Zimmerberg J. Cytotoxicity mediated by the Fas ligand (FasL)-activated apoptotic pathway in stem cells. J Biol Chem. 2009;284:22022-22028.
- Bezrukov L, Blank PS, Polozov IV, Zimmerberg J (2009). An adhesion-based method for plasma membrane isolation: Evaluating cholesterol extraction from cells and their membranes. Anal Biochem. 2009;394:171-6.
- Stenkula KG, Lizunov VA, Cushman SW, Zimmerberg J. Insulin controls the spatial distribution of GLUT4 on the cell surface through regulation of its postfusion dispersal. Cell Metab. 2010;12:250-9.
- Glushakova S, Humphrey G, Leikina E, Balaban A, Miller J, Zimmerberg J. New stages in the program of malaria parasite egress imaged in normal and sickle erythrocytes. Curr Biol. 2010;20:1117-21.
- Frolov VA, Zimmerberg J. Cooperative elastic stresses, the hydrophobic effect, and lipid tilt in membrane remodeling. FEBS Lett. 2010;584:1824-9.
- Shnyrova AV, Frolov VA, Zimmerberg J. Domain-driven morphogenesis of cellular membranes. Curr Biol. 2009; 19:R772-80.
- Shnyrova AV, Zimmerberg J. Chapter 4 - Reconstitution of membrane budding with unilamellar vesicles. Methods Enzymol. 2009;464:55-75.
- Fitzgerald W, Chen S, Walz C, Zimmerberg J, Margolis L, Grivel JC. Immune suppression of human lymphoid tissues and cells in rotating suspension culture and onboard the International Space Station. In Vitro Cell Dev Biol Anim. 2009;45:622-632.
- Lizunov VA, Lisinski I, Stenkula K, Zimmerberg J, Cushman SW. Insulin regulates fusion of GLUT4 vesicles independent of Exo70-mediated tethering. J Biol Chem. 2009;284:7914-9.
Collaborators
- Samuel W. Cushman, PhD, Diabetes Branch, NIDDK, Bethesda, MD
- Klaus Gawrisch, PhD, Laboratory of Membrane Biochemistry and Biophysics, NIAAA, Bethesda, MD
- Samuel T. Hess, PhD, University of Maine, Orono, ME
- Thomas S. Reese, MD, Laboratory of Neurobiology, NINDS, Bethesda, MD
- Sandra Schmid, PhD, The Scripps Research Institute, La Jolla, CA
Contact
For more information, email zimmerbj@mail.nih.gov.