You are here: Home > Section on Cell Biophysics
Cell Biophysics
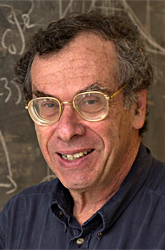
- Ralph Nossal, PhD, Head, Section on Cell Biophysics
- Dan Sackett, PhD, Staff Scientist
- Anand Banerjee, PhD, Visiting Fellow
- Raimon Sunyer, PhD, Visiting Fellow
- Silviya Zustiak, PhD, Postdoctoral Fellow
- Matthew Mirigian, BA, Postbaccalaurate Fellow
- Hacène Boukari, PhD, Guest Researcher
- Jennifer Galanis, MD, Guest Researcher
- Norman Gershfeld, PhD, Guest Researcher
- Ji-Youn Lee, PhD, Guest Researcher
We perform studies on cell behavior that can be linked to underlying physical mechanisms, for which we develop and apply methodologies based on mathematical and physical principles. Our research also involves biochemical and cell-biological techniques. Projects currently include (i) constructing a physical model to explain the stochastic nature of coated vesicle biogenesis during receptor-mediated endocytosis, (ii) determining the mechanical properties of clathrin cages and using that knowledge to gain insight into membrane transformations that occur during vesicle trafficking, (iii) exploring how substrate mechanical properties affect the movements of locomoting eukaryotic cells, and (v) understanding how certain small molecules interact with microtubules and thereby act as antimitotic agents. We also develop new experimental modalities to characterize these and related phenomena. We have a particular interest in the ways cellular activities are coordinated in space and time.
Biophysical methods and models
We perform studies on cell behavior that can be linked to underlying physical mechanisms, for which we develop and apply methodologies based on mathematical and physical principles. Our research also involves biochemical and cell-biological techniques. Projects currently include (i) constructing a physical model to explain the stochastic nature of coated vesicle biogenesis during receptor-mediated endocytosis, (ii) determining the mechanical properties of clathrin cages and using that knowledge to gain insight into membrane transformations that occur during vesicle trafficking, (iii) exploring how substrate mechanical properties affect the movements of locomoting eukaryotic cells, and (v) understanding how certain small molecules interact with microtubules and thereby act as antimitotic agents. We also develop new experimental modalities to characterize these and related phenomena. We have a particular interest in the ways cellular activities are coordinated in space and time.
Biophysical methods and models
We have continued to develop physics-based methodologies for the investigation of complex biological structures and materials. We use well controlled models to gain insights that may subsequently be useful in inquiries of specific phenomena of biomedical import.
One of our activities has involved studying the effects of multiple scattering on the interpretation of fluorescence correlation spectroscopy (FCS) measurements performed on optically dense samples. A unique power of FCS is that it can detect the motions of fluorescent entities while signals from non-fluorescent surroundings can be ignored. For this reason, FCS is being used increasingly to study particles located in complex milieu; examples are molecules moving on cell surfaces, antibiotics and viruses diffusing in biofilms, and morphogens moving within embryos. Using well defined scattering models, we investigated the reliability of parameters determined by FCS when it is used to probe molecules in such environments. For example, we measured FCS auto-correlation functions of Atto 488 dye molecules diffusing in solutions of polystyrene beads, which act as scatterers. As much as a two-fold, scattering-linked increase in the illuminated volume was noted, but only minimal increase in apparent diffusivity was evident. We employed Monte-Carlo simulations to analyze the illuminated beam profile, finding that beam broadening occurs primarily along the axial direction. However, the increased volume in the axial direction has only a negligible effect on the measured diffusion time because intensity fluctuations arising from diffusion in the radial direction are dominant in FCS measurements. Although a reduction of the incident intensity happens at the focal point, our results indicate that significant measurement artifacts do not occur, implying that single-photon FCS can be used to probe diffusivity in optically dense media, when sufficient signal intensity is attainable. (A paper based on this study is under review.) The results have informed work in which we employ FCS to investigate the diffusion of macromolecules and other targets within the fluid phase of polymer gels and in concentrated polymer solutions. Again making use of the ability of FCS to distinguish the movements of fluorescent particles from those of a non-fluorescent background, we have been studying various physical properties including the link between diffusion and such variables as particle size and degree of crosslinking of the polymer-chain constituents.
In addition, we collaborated on two projects aimed at developing new, low-cost, optical imaging methods for biological samples. One involves constructing an instrument to perform rapid, full-field polarization fluorescence microscopy. Our scheme utilizes a quarter-wave plate in combination with a liquid crystal variable retarder (LCVR) to provide a tunable method to rotate polarization of light prior to its being coupled into a fluorescence microscope. We demonstrated the efficacy of this method by observing localized molecular orientations of probes attached to lipid membranes (1). In principle, one can use such instrumentation to study any cellular process that involves changes in molecular orientation, as long as appropriate fluorescent labels can be found. The second activity supports the development and use of hyperspectral imaging to characterize the spatial organization of complex, multispecies cellular communities. Microarray phantoms containing several dyes were fabricated and used to test image analysis algorithms for this process.
Complex systems biophysics
We have been employing physical and mathematical methods to understand the biophysics of complex cellular processes. In particular, we studied the biogenesis of coated vesicles involved in clathrin-mediated endocytosis (CME) and other intracellular transport processes. CME is the principal pathway for the regulation of receptors, and internalization of certain nutrients and signaling molecules, at the plasma membrane of eukaryotic cells. The early stage of receptor-mediated endocytosis involves the formation of transient structures known as clathrin-coated pits (CCPs) which, depending on the detailed energetics of protein binding and associated membrane transformations, either mature into clathrin-coated vesicles (CCVs) or regress and vanish from the cell surface. The former are referred to as "productive" CCPs and the latter as "abortive" CCPs. We developed a simple physical model for CCP dynamics and carried out Monte Carlo simulations to investigate the time development of CCP size and explain the origin of abortive pits and features of their lifetime distribution. By fitting the results of the simulations to experimental data, we were able to estimate values of the free energy changes involved in formation of the clathrin-associated protein complexes that constitute the coat, and we showed how the binding of cargo might modify the coat parameters and thereby facilitate CCV formation (2). We also derived analytical expressions for the lifetime distribution and the distribution of maximum sizes of abortive pits, which may be useful in extracting additional information about the mechanism of CCP assembly from experimental data. Moreover, we obtained a mathematical expression for the stochastic fate of a nascent pit, i.e., whether it will disassemble or mature into CCVs. This expression is being used to identify parameters that affect particular processes in which CME plays a role, especially those of nanoparticles that are employed as drug delivery vehicles.
Also, we are using atomic force microscopy (AFM) and single-molecule force spectroscopy (SMFS) to characterize intermolecular interactions and domain structures of clathrin triskelions and clathrin assemblies. To assess triskelion structure and triskelion-triskelion interactions, we subject purified individual triskelions, bovine-brain CCVs, and reconstituted clathrin–AP180 coats to AFM–SMFS pulling experiments and apply newly derived analytics to extract force-extension relations from very large data sets. For individual triskelions, SMFS reveals a series of unfolding events associated with individual heavy chain alpha-helix hairpins containing about 30 amino acid residues. Cooperative unraveling of several hairpin domains up to the size of the known repeating motif of about 145 amino acid residues is also seen. We find that the clathrin lattices of AP180–mediated coats are energetically easier to unravel than those of native CCVs. The spectroscopic fingerprinting characteristics for single triskelions, clathrin–AP180 coats and CCVs vary noticeably and reveal non-trivial dependence on the force loading rate. Studies of clathrin assemblies expose weaker, but coordinated, clathrin-clathrin interactions that are indicative of the inter-leg associations essential for CME. (A paper based on this work is undergoing review.) In a related study, we have been using quartz crystal microbalance-dissipation (QCM-D) instrumentation to investigate the mesoscopic mechanical properties of the triskelions. We find that the apparent shear moduli of these structures are approximately one to two orders of magnitude smaller than the Young's modulus of a triskelion leg. The values of these shear moduli vary strongly with buffer properties and also depend, to a lesser degree, on properties of the substrate upon which a triskelion rests.
In a separate project, also related to mechanical aspects of cell response, we are establishing a reliable method for assessing the coupling between substrate properties and fundamental cell processes such as angiogenesis, neurogenesis, and cancer metastasis, which are thought to be modulated by extracellular matrix stiffness. The availability of matrix substrates having well defined stiffness profiles can be of great importance in biophysical studies of cell-substrate interaction. We thus developed a method to fabricate bio-compatible hydrogels with a well defined linear stiffness gradient. This method, involving photopolymerization of films by progressively uncovering an acrylamide/bis-acrylamide solution initially covered with an opaque mask, can be easily implemented with common lab equipment. It produces linear stiffness gradients of at least 40 kPa/mm, extending from <1 kPa to 80 kPa (in units of shear modulus). Hydrogels with less steep gradients and narrower stiffness ranges can easily be produced. The hydrogels can be covalently functionalized with uniform coatings of proteins that promote cell adhesion. Cell spreading on these hydrogels linearly correlates with hydrogel stiffness, indicating that this technique can be used to study the mechanical response of living cells (3). An extension of this work will focus on understanding the effects of substrate rigidity on the collective movements of mechanically interacting cells. Such work may have applications in studies of wound healing, cancer metastasis, and normal and aberrant development of embryonic tissues.
Finally, we are developing a "high-throughput" polyacrylamide (PA)-based stiffness assay, which can be used to study how matrix stiffness affects cell fate. The gels were coated with collagen in order to facilitate cell attachment and proliferation. Polyacrylamide is the material of choice because it spans stiffness values from 0.3 to 300 kPa. The high-throughput format was chosen in order to facilitate obtaining dose response curves and to provide for simultaneous testing of several parameters. The assay is an improvement upon other techniques in terms of preparation time, robustness, and cost. This PA–based assay is currently being used to test the effect of stiffness on cancer cell responsiveness to anti-cancer drugs. In particular, we are testing several cell lines for their susceptibility to microtubule-targeting agents.
Tubulin polymers and cytoskeletal organization
We continued our study on the properties of microtubules (MT) and of drugs that alter these properties. As described below, our recent work identified the binding site for a new microtubule-stabilizing drug, peloruside, permitting an understanding of an observed synergy in drug action when it is combined with established microtubule-stabilizing drugs. In addition, we examined the clinical activity of microtubule-active drugs and inferred that their activity against human tumors is likely not attributable to their activity as anti-mitotic agents.
The microtubule-stabilizing compounds Taxol and epothilone have achieved significant clinical success against a variety of human tumors. Consequently new compounds that share this mechanism are being sought in the hope that they may avoid some of the side effects of Taxol and epothilones. Two new compounds that share the mechanism of microtubule stabilization are peloruside and laulimalide. Surprisingly, peloruside or laulimalide act synergistically when combined with Taxol or epothilone, but not when combined with each other, suggesting that the binding site and detailed molecular mechanism for the newer compounds differ from those of the established drugs. We previously localized the binding region for peloruside to beta tubulin, using hydrogen-deuterium exchange mass spectrometry (4). More recently, we succeeded in defining this binding site in detail by mapping mutations in beta tubulin that confer resistance to the cytotoxic activity of peloruside. The mutations cluster in the surface-exposed pocket on beta tubulin that was implicated from our mass spectrometry studies. Molecular modeling of the peloruside-tubulin interaction indicates that the side chain on the drug fits into the deepest part of the binding pocket, where the mutations are located. The binding pocket is significantly removed from the location of the binding site for Taxol. These combined results provide a basis for understanding the synergy observed between the two drugs.
The established clinical activity of microtubule-stabilizing drugs such as Taxol and the epothilones (as well as the activity of microtubule-destabilizing drugs such as vincristine) against human cancers is usually thought to be attributable to their activity against mitosis. There is no doubt that the drugs inhibit mitosis. However, this explanation alone seems incompatible with the observation that the growth rate of most human tumors is very slow, certainly slower than the growth rates of cells in culture on which the observations of mitotic inhibition are made. We compiled the results of a large number of clinical trials of compounds whose activity is restricted to mitosis (unlike microtubule-targeting drugs, whose target is present throughout the cell cycle). The mitosis-specific drugs target kinases that only are produced and active during mitosis. The compounds inhibit mitosis in cell cultures just as well as do anti-microtubule drugs. However, they show essentially no clinical activity against human tumors. We argue that this supports the view that microtubule-targeting drugs owe their clinical activity to non-mitotic targets, even though they will inhibit mitosis if it occurs during drug treatment (5). This provides a basis for identifying microtubule-dependent target mechanisms in cells that are not undergoing mitosis.
Additional Funding
- Joint NIST-NIH National Research Council Postdoctoral Fellowship (2010-2012) to Ji Youn Lee (concluded)
- Catalan Government Award (Spain, 2011-2012) to Raimon Sunyer (concluded)
Publications
- Lesoine JF, Lee JY, Krogmeier JR, Kang H, Clarke ML, Chang R, Sackett DL, Nossal R, Hwang J. Quantitative scheme for full-field polarization rotating fluorescence microscopy using a liquid crystal variable retarder. Rev Sci Instrum 2012;83:053705.
- Banerjee A, Berezhkovskii A, Nossal R. Stochastic model of clathrin-coated pit assembly. Biophys J 2012;102:2725-2730.
- Sunyer R, Jin AJ, Nossal RJ, Sackett DL. Fabrication of hydrogels with steep stiffness gradients for studying cell mechanical response. PLoS One 2012;7:e46107.
- Begaye A, Trostel S, Zhao Z, Taylor RE, Schriemer DC, Sackett DL. Mutations in the β-tubulin binding site for peloruside A confer resistance by targeting a cleft significant in side chain binding. Cell Cycle 2011;10:3387-3396.
- Komlodi-Pasztor E, Sackett DL, Wilkerson J, Fojo T. Mitosis is not a key target of microtubule agents in patient tumors. Nat Rev Clin Oncol 2011;8:244-250.
Collaborators
- Susan Bane, PhD, Binghamton University, Binghamton, NY
- Alexander Berezhkovskii, PhD, Division of Computational Bioscience, CIT, NIH, Bethesda, MD
- Hacène Boukari, PhD, Delaware State University, Dover, DE
- Tito Fojo, MD, PhD, Medical Oncology Branch, NCI, Bethesda, MD
- Amir Gandjbakhche, PhD, Program in Pediatric Imaging and Tissue Sciences, NICHD, Bethesda, MD
- Jeeseong Hwang, PhD, National Institute of Standards and Technology, Gaithersburg, MD
- Albert J. Jin, PhD, Laboratory of Cellular Imaging and Macromolecular Biophysics, NIBIB, Bethesda, MD
- Eileen Lafer, PhD, University of Texas Health Science Center, San Antonio, TX
- Ji-Youn Lee, PhD, National Institute of Standards and Technology, Gaithersburg, MD
- John Lemoine, PhD, National Institutes of Standards and Technology, Gaithersburg, MD
- Naomi Morrissette, PhD, University of California Irvine, Irvine, CA
- John Park, MD, Surgical Neurology Branch, NINDS, Bethesda, MD
- Jason Riley, PhD, Program on Pediatric Imaging and Tissue Science, NICHD, Bethesda, MD
- Dave Schriemer, PhD, University of Calgary, Calgary, Canada
- David Sept, PhD, University of Michigan, Ann Arbor, MI
- Richard Taylor, PhD, Notre Dame University, Notre Dame, IN
- Karl Werbovetz, PhD, Ohio State University, Columbus, OH
- Al Yergey, PhD, Mass Spectrometry Core Facility, NICHD, Bethesda, MD
Contact
For more information, email nossalr@mail.nih.gov.