You are here: Home > Section on Molecular Transport
Biophysics of Large Membrane Channels
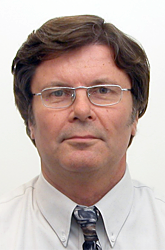
- Sergey M. Bezrukov, PhD, Head, Section on Molecular Transport
- Tatiana K. Rostovtseva, PhD, Staff Scientist
- Philip A. Gurnev, PhD, Research Fellow
- Oscar Teijido Hermida, PhD, Visiting Fellow
- Shay M. Rappaport, PhD, Visiting Fellow
- Kely L. Sheldon, MS, Predoctoral Intramural Research Training Award Fellow
Combining experiments on channel reconstitution into planar lipid bilayers with physical theory, we study mitochondrial and bacterial membrane proteins that produce "large" beta-barrel channels. These channel-forming proteins are not only the gateways to metabolite exchange between different cellular compartments and cells, they are also recognized as multifunctional membrane receptors and components of many toxins, which are beginning to emerge as novel drug targets. This line of research serves as the basis for the development of new approaches to the treatment of various diseases, for which regulation of transport through ion channels plays a key role.
The channel-forming proteins we work with include the VDAC (Voltage-Dependent Anion Channel) from the outer membrane of mitochondria, OmpF (general bacterial porin from Escherichia coli), LamB (sugar-specific bacterial porin from Escherichia coli), OprF (porin from Pseudomonas aeruginosa), translocation pores of B. anthracis, C. botulinum, and C. perfringens binary toxins, Epsilon toxin (from Clostridium perfringens), alpha-Hemolysin (toxin from Staphylococcus aureus), Alamethicin (amphiphilic peptide toxin from Trichoderma viride), Syringomycin E (lipopeptide toxin from Pseudomonas syringae), and the bacterial peptide TisB involved in persister cell biofilm formation. To study these channels under precisely controlled conditions, we first isolate the channel-forming proteins from their host organisms, purify them, and then reconstitute them into planar lipid bilayer membranes. Our main goal is to elucidate the physical principles and molecular mechanisms that control metabolite flux under normal and pathological conditions. Specifically, we study channel interactions with the lipid membrane as modified by volatile anesthetics, with cytosolic proteins, and with newly synthesized drugs, such as blockers of the translocation pores of bacterial toxins. By learning the physics, chemistry, and physiology of channel functioning, we strive to determine how to design new agents and strategies that effectively correct the deviant interactions associated with disease.
The inhalational anesthetic halothane changes the domain structure of a binary lipid membrane
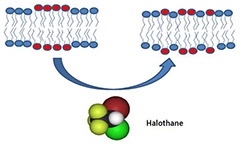
Click image to enlarge.
Figure 1
X-ray and neutron diffraction studies of a binary lipid membrane demonstrate that halothane at physiological concentrations produces a pronounced redistribution of lipids between domains of different lipid types identified by different lamellar d-spacings and isotope composition.
The molecular mechanisms of volatile anesthetic action remain obscure despite a long history of research and clinical use for over a century and a half. The remarkable correlation between anesthetic solubility in oil and anesthetic potency, the so-called Meyer-Overton rule, strongly suggests a lipid membrane–mediated mechanism. Paradoxically, structural studies of lipid bilayer membranes demonstrated negligible effects of the presence of anesthetics. Thus, in classic experiments of 30 years ago, employing X-ray and neutron diffraction from dimyristoylphosphatidylcholine (DMPC)/cholesterol membranes, Franks and Lieb found that for inhalation anesthetics "…at surgical concentrations, however, there are no significant changes in bilayer structure." Since then, the conceptual view of cell membranes has shifted from relatively homogeneous lipid bilayers with interspersed proteins to complex lipid mixtures, with laterally separated membrane domains formed as a result of lipid demixing. Accumulating evidence indicates that certain membrane proteins are clustered in domains such as cholesterol-rich "lipid rafts." Guided by this conceptual shift and jointly with NIST scientists, we performed X-ray and neutron diffraction experiments on a binary lipid membrane. Specifically, we studied a 1:1 mixture of dipalmitoylphosphatidylcholine (DPPC) and dilauroylphaphatidylcholine (DLPC) to demonstrate that halothane, but not dichlorohexafluorocyclobutane, a halogenated nonanesthetic of similar properties, produces a pronounced redistribution of lipids between different domains at physiologically relevant concentrations (Figure 1). The domains of different lipid types were identified through their distinct lamellar d-spacings and isotope composition. The results demonstrate a specific effect of inhalational anesthetics on mixing phase equilibria. Combined with a growing body of data revealing that conformational dynamics of transmembrane proteins are highly sensitive to the parameters of the lipid bilayer within which they reside, our findings suggest that halothane and other volatile anesthetics may act through the cell membrane by changing its domain structure (1). We hope that these results are of ultimate help in the search for more potent and safe anesthetizing agents.
VDAC inhibition by tubulin and its physiological implications
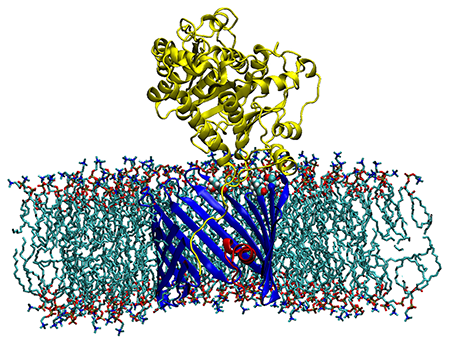
Click image to enlarge.
Figure 2. Sagittal section of the alpha-tubulin-VDAC complex in a DOPC lipid bilayer
The membrane-inserted VDAC1 is shown in blue with its N-terminal in red. The membrane-tethered alpha-tubulin is yellow. T165, T188, and T212 residues of VDAC1 forming stable H-bonds to alpha-tubulin are shown as ball-and-stick. Low-energy representative complex coordinates were obtained with ROSETTA protein-protein docking using a combination of Monte-Carlo modeling and rigid-body rotation/translations with 2,500 trial iterations.
Recently, we discovered that the abundant cytoskeletal protein tubulin is an important player in the regulation of the Voltage-Dependent Anion Channel (VDAC) of the outer mitochondrial membrane. We have now extended our study of tubulin–VDAC interaction in three directions. First, we investigated the structural features of the blocked state as revealed by its sensitivity to the charge in the membrane surface. We found that the relative residual conductance of the tubulin-blocked state of VDAC is sensitive to the surface charge of the membrane, thus confirming the tail-in-the-pore model of blockage that we had suggested earlier (Figure 2). Second, we demonstrated that in vitro phosphorylation of VDAC by either glycogen synthase kinase-3beta or cAMP–dependent protein kinase A raises the on-rate of tubulin binding to VDAC by orders of magnitude. Experiments on HEPG2 human hepatoma cells supported our conjecture that dimeric tubulin and channel phosphorylation regulate VDAC permeability for mitochondrial respiratory substrates. Treatment of HepG2 cells with colchicine prevented microtubule polymerization, thus increasing dimeric tubulin availability in the cytosol, which leads to a reduction in mitochondrial potential, as measured by assessing mitochondrial tetramethylrhodamine methylester uptake with confocal microscopy. Third, we demonstrated that a critical step in the mechanism of VDAC blockage by tubulin is tubulin interaction with the membrane. The on-rate of the blockage varies up to 100-fold, depending on the lipid composition used for bilayer formation in reconstitution experiments (2, 3). Thus, in addition to revealing an important step in the tubulin-VDAC interaction, our results provide a novel example of a lipid-controlled protein-protein interaction in which the choice of lipid species is able to change the equilibrium binding constant by orders of magnitude.
Immediate physiological implications of these findings include new insights into cell signaling pathways and cytoskeleton/microtubule activity in health and disease, especially in the case of the highly dynamic microtubule network characteristic of carcinogenesis and cell proliferation. Our findings may help to identify new mechanisms of the mitochondria-associated action of chemotherapeutic microtubule-targeting drugs, and also to understand why and how cancer cells preferentially use inefficient glycolysis rather than oxidative phosphorylation (Warburg effect).
Physical theory of channel-facilitated transport
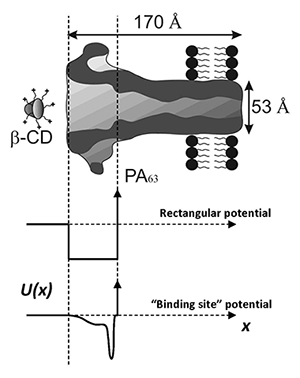
Click image to enlarge.
Figure 3
A cartoon illustrating two hypothetical profiles of the potential of mean force chosen for the thermodynamic analysis of multi-charged blocker interaction with the anthrax's PA63 channel. The vertical dashed lines indicate the part of the channel lumen accessible to the blocker molecule.
Further development of the physical theory of channel-facilitated transport is required for a deeper understanding of its regulation mechanisms. This year, we concentrated on two topics: the effects of clustering and the thermodynamics of channel blockage.
Clustering of receptors, transporters, and ion channels, which appears to be the rule rather than the exception, complicates the quantitative description of transport in biological systems. We analyzed the effects of clustering by considering an aggregate of absorbing disks on an otherwise reflecting wall. Trapping of diffusing particles by such an aggregate is a manifestly many-body problem because of the competition between disks for the particles. By replacing the cluster with an effective uniformly absorbing spot, we derived a simple algebraic expression for the rate constant that characterizes the trapping. The formula shows how the rate constant depends on the size, shape, and the density of packing in the cluster (4). The obtained analytical results may provide a rationale for surface clustering on plasma membranes in addition to the usual explanation of enhancing two-dimensional macromolecular interaction.
The thermodynamics of binding reactions is usually studied in the framework of the linear van't Hoff analysis of the equilibrium constant temperature dependence. The logarithm of the equilibrium constant is plotted against inverse temperature to discriminate between the two terms: an enthalpic contribution that is linear in the inverse temperature, and a temperature-independent entropic contribution. When we apply this approach to a particular case—blockage of the anthrax PA63 channel by a multi-charged cyclodextrin derivative—we obtain nearly linear behavior with a slope that is characterized by enthalpy of about 1 kcal/mol. In contrast, from blocker partitioning between the channel and the bulk, we estimate the depth of the potential well for the blocker in the channel to be at least 8 kcal/mol. To understand this apparent discrepancy, we used a simple model of particle interaction with the channel (Figure 3) and showed that the significant difference between the two estimates can be attributed to the temperature dependence of the physical forces between the blocker and the channel. In particular, we demonstrated that, if the major component of blocker-channel interaction is van der Waals interactions and/or Coulomb forces in water, the van't Hoff enthalpy of the binding reaction may be close to zero or even negative, even in cases of relatively strong binding. The results are quite general and, therefore, of importance to studies of enzymatic reactions, rational drug design, small-molecule binding to proteins, protein-protein interactions, protein folding, and others (5).
Additional Funding
- NIAID intramural biodefense grant (2009-2011) "From anthrax to botulism, cholera, and epsilon toxin: in search for broad-spectrum antitoxins that block virulent nanopores"
Publications
- Weinrich M, Nanda H, Worcester DL, Majkrzak CF, Maranville BB, Bezrukov SM. Halothane changes the domain structure of a binary lipid membrane. Langmuir 2012;28:4723-4728.
- Rostovtseva TK, Gurnev PA, Chen M-Y, Bezrukov SM. Membrane lipid composition regulates tubulin interaction with mitochondrial voltage dependent anion channel. J Biol Chem 2012;287:29589-29598.
- Rostovtseva TK, Bezrukov SM. VDAC interaction with tubulin and its physiological implications. Biochim Biophys Acta 2012;1818:1526-1535.
- Berezhkovskii AM, Dagdug L, Lizunov VA, Zimmerberg J, Bezrukov SM. Clusters of absorbing disks on a reflecting wall: competition for diffusing particles. J Chem Phys 2012;136:211102.
- Nestorovich EM, Karginov VA, Berezhkovskii AM, Parsegian VA, Bezrukov SM. Kinetics and thermodynamics of binding reactions as exemplified by anthrax channel blockage with a cationic cyclodextrin derivative. Proc Natl Acad Sci USA 2012;109:18453–18458.
Collaborators
- Vicente M. Aguilella, PhD, Universidad Jaume I, Castellón, Spain
- Alexander M. Berezhkovskii, PhD, Division of Computational Bioscience, CIT, NIH, Bethesda, MD
- Susan K. Buchanan, PhD, Laboratory of Molecular Biology, NIDDK, Bethesda, MD
- Leonid V. Chernomordik, PhD, Program in Physical Biology, NICHD, Bethesda, MD
- Leonardo Dagdug, PhD, Universidad Autónoma Metropolitana-Iztapalapa, Mexico City, Mexico
- Vladimir A. Karginov, PhD, Innovative Biologics, Herndon, VA
- John J. Lemasters, PhD, Medical University of South Carolina, Charleston, SC
- Ekaterina M. Nestorovich, PhD, The Catholic University of America, Washington, DC
- Sergei Y. Noskov, PhD, University of Calgary, Canada
- Adrian Parsegian, PhD, University of Massachusetts Amherst, Amherst, MA
- Dan Sackett, PhD, Program in Physical Biology, NICHD, Bethesda, MD
- Gerhard Wagner, PhD, Harvard Medical School, Boston, MA
- Michael Weinrich, MD, National Center for Medical Rehabilitation Research, NICHD, Bethesda, MD
- David L. Worcester, PhD, National Institute of Standards and Technology. Gaithersburg, MD
- Josh Zimmerberg, MD, PhD, Program in Physical Biology, NICHD, Bethesda, MD
Contact
For more information, email bezrukov@helix.nih.gov or visit smt.nichd.nih.gov.