You are here: Home > Section on Gamete Development
Cell Cycle Regulation in Oogenesis

- Mary Lilly, PhD, Head, Section on Gamete Development
- John Reich, PhD, Postdoctoral Fellow
- Tanveer Akbar, PhD, Visiting Fellow
- Weili Cai, PhD, Visiting Fellow
- Youheng Wei, PhD, Visiting Fellow
- Kuikwon Kim, MS, Technician
The long-term goal of our laboratory is to understand how the cell-cycle events of meiosis are coordinated with the developmental events of gametogenesis. Chromosome mis-segregation during female meiosis is the leading cause of miscarriages and birth defects in humans. Recent evidence suggests that many meiotic errors occur downstream of defects in oocyte growth and/or the hormonal signaling pathways that drive differentiation of the oocyte. Thus, an understanding of how meiotic progression and gamete differentiation are coordinated during oogenesis is essential to studies in both reproductive biology and medicine. We use the genetically tractable model organism Drosophila melanogaster to examine how meiotic progression is both coordinated with and instructed by the developmental program of the egg.
In mammals, studies on the early stages of oogenesis face serious technical challenges in that entry into the meiotic cycle, meiotic recombination, and the initiation of the highly conserved prophase I arrest all occur during embryogenesis. In contrast, in Drosophila these critical events of early oogenesis all take place continuously within the adult female. Easy access to the early stages of oogenesis, coupled with available genetic and molecular genetic tools, makes Drosophila an excellent model for studies on meiotic progression and oocyte development.
To understand the regulatory inputs that control early meiotic progression, we are working to determine how the oocyte initiates and then maintains the meiotic cycle within the challenging environment of the ovarian cyst. Our studies focus on questions that are relevant to the development of all animal oocytes. What strategies does the oocyte use to protect itself from inappropriate DNA replication? How does the oocyte inhibit mitotic activity before meiotic maturation and the full growth and development of the egg? How does cell-cycle status within the ovarian cyst influence the differentiation of the oocyte? To answer these questions, we have undertaken studies to determine the basic cell-cycle program of the developing ovarian cyst.
The Mio/Seh1 complex regulates meiotic progression and metabolism during oogenesis (Figure 1).
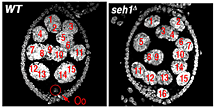
Click image to enlarge.
Figure 1. The nucleoporin Seh1 is required for maintenance of the meiotic cycle.
Wild-type (WT) and seh1Δ egg chambers stained with the DNA dye DAPI. The seh1Δ egg chamber has 16 polyploid nurse cells but no oocyte (Oo).
The pathways that control entry into the meiotic cycle and early meiotic progression are poorly understood in metazoans. We previously identified a gene, missing oocyte (mio), that regulates nuclear architecture and meiotic progression in early ovarian cysts. The mio gene is highly conserved from yeast to humans. In mio mutants, the oocyte enters the meiotic cycle and progresses to pachytene. The meiotic state, however, is not maintained. Ultimately, mio oocytes withdraw from meiosis, enter the endocycle, and become polyploid. Genetic analysis indicates that mio acts early in oogenesis, before the formation of the synaptonemal complex (SC) and meiotic recombination. Moreover, mio interacts with genes that control DNA metabolism. Therefore, mio provides an excellent entry point to explore how the unique cell biology of the early ovarian cyst and the establishment of the meiotic program influence the downstream events of oocyte differentiation and meiotic progression.
To understand more fully the role of mio in oogenesis, we initiated a series of experiments to identify additional proteins that function in the Mio pathway. From these studies, we determined that Mio is present in a large multi-protein complex containing at least four additional proteins, including Mtc5, Iml1, Npr3, and the structural nucleoporin Seh1. Seh1 is a subunit of the Nup107?160 complex, the major structural subcomplex of the nuclear pore complex (NPC). Like mio, seh1 has a critical germ line function during oogenesis. Intriguingly, we demonstrated that Mio does not associate with other nucleoporins, suggesting that Mio and Seh1 may define a novel complex independent of the NPC. In both mio and seh1 mutants, a fraction of oocytes fail to maintain the meiotic cycle and develop as pseudo-nurse cells. seh1 oocytes that maintain the meiotic cycle display meiosis I spindle abnormalities, strongly suggesting a primary defect in the initiation of meiotic recombination. We find that, in addition to a physical interaction, mio and seh1 genetically interact. Specifically, seh1 acts as a strong dominant suppressor of the mio ovarian phenotype. Surprisingly, our characterization of a seh1 null allele indicated that, while required in the female germ line, seh1 is dispensable for the development of somatic tissues. Our work represents the first examination of Seh1 function within the context of a multi-cellular organism. In summary, our studies demonstrate that Mio is a novel interacting partner of the conserved nucleoporin Seh1 and add to the growing body of evidence that structural nucleoporins can have novel tissue-specific roles.
Recent reports indicate that, in yeast, as we observe in Drosophila, Mio and Seh1 are present in a large multi-protein complex. This newly discovered?complex has been named the SEA complex (Seh-1 interacting complex). SEA complex members have been implicated in the regulation of the response to amino acid starvation and membrane trafficking. In addition, several members of the SEA complex localize to the vacuole. We determined that, in Drosophila, as is observed in yeast, mio mutants exhibit defects in metabolic regulation. Currently we are working to define the role of the SEA complex in meiotic progression, growth control, and membrane dynamics.
Wee1 regulates Cdk1 activity and nuclear organization in prophase I oocytes (Figure 2).
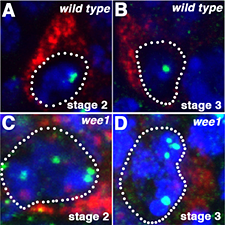
Click image to enlarge.
Figure 2. wee1 oocytes have abnormal chromatin organization.
(A, C) Wild-type and (B, C) wee1 oocytes stained with DAPI and antibodies against the centromere protein Cid (green) and the oocyte marker Orb (red). In wee1 oocytes, the centromeres frequently fail to cluster.
We determined that the Wee1 kinase, in order to?maintain low Cdk1 activity in prophase I oocytes,?acts in parallel to pathways that restrict Cyclin accumulation. In our previous work, we reported that, that during Drosophila oogenesis, the APC/CFzr/Cdh1 and the translational repressor Bruno (aret) both prevent inappropriate accumulation of the mitotic cyclin Cyclin A in early ovarian cysts. Cyclin A is an activating subunit of the mitotic kinase Cdk1. However, our studies strongly suggested that an additional pathway ensures low Cdk1 activity in prophase oocytes independent of Cyclin A accumulation. We reasoned that members of the Wee1-like kinase family were good candidates for this alternative pathway.
Activation of Cdk1 kinase requires association with a mitotic Cyclin as well as the dephosphorylation of Thr 14 and Tyr 15 residues on the kinase. The phosphorylation of these two key residues is controlled by the activity of the Wee1 family of kinases. In mammals, several Wee1 family members inhibit Cdk1 activity in prophase I oocytes. In Drosophila, there are two well characterized Wee1 kinase family members: Myt1 and Wee1. Loss of Myt1 results in elevated non-disjunction during Drosophila oogenesis, implicating Myt1 as a regulator of female meiosis. However, consistent with published results, we find that myt1 mutants do not exhibit altered nuclear structure or other defects in the early oocyte. In contrast, when we examined ovaries from homozygous and trans-heterozygous wee1 females, we discovered defects in DNA conformation and SC structure. Specifically, in a fraction of wee1 oocytes, we observed premature disassembly of the SC in stage 2 of oogenesis, a decrease in centromere clustering, and a delay in karyosome formation. The phenotypes are strongly enhanced by mutations in genes such as aret that inhibit the accumulation of the mitotic cyclins. Recent evidence indicates that, in Drosophila, Wee1 phosphorylates g-TuRC and the kinesin 5 family member Klp61. Therefore, we performed a series of experiments to confirm that the early oocyte phenotype observed in wee1 mutants is attributable to an unscheduled increase in Cdk1 activity rather than to Wee1 acting on an alternative target. Tribbles regulates the degradation of String and Twine, two Cdc25-like phosphatases that oppose the Wee1-dependent inhibitory phosphorylation of Cdk1. We find that mutants in tribbles recapitulate the early oocyte phenotypes of wee1 mutants. The data suggest that the observed phenotypes result from the action of Wee1 on Cdk1 and not an alternative target. In summary, we demonstrated that prophase I oocytes utilize several semi-redundant pathways to prevent the inappropriate activation of Cdk1 in early prophase I oocytes.
Publications
- Senger S, Csokmay J, Akbar T, Jones TI, Sengupta P, Lilly MA. The nucleoporin Seh1 forms a complex with Mio and serves an essential tissue-specific function in Drosophila oogenesis. Development 2011;138:2133-2142.
- Mitra K, Rikhy R, Lilly M, Lippincott-Schwartz J. DRP1-dependent mitochondrial fission initiates follicle cell differentiation during Drosophila oogenesis. J Cell Biol 2012;197:487-497.
Collaborators
- Jennifer Lippincott-Schwartz, PhD, Cell Biology and Metabolism Program, NICHD, Bethesda, MD
- Kim McKim, PhD, Waksman Institue of Microbiology, Rutgers, The State University of NJ, Piscataway, NJ
- Juan Riesgo-Escovar, PhD, Universidad Nacional Autónoma de México, Querétaro, Mexico
Contact
For more information, email mlilly@helix.nih.gov or visit irp.nih.gov/pi/mary-lilly.