You are here: Home > Section on Molecular Transport
Biophysics of Large Membrane Channels
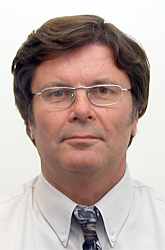
- Sergey M. Bezrukov, PhD, Head, Section on Molecular Transport
- Tatiana K. Rostovtseva, PhD, Staff Scientist
- Oscar Teijido Hermida, PhD, Visiting Fellow
- David P. Hoogerheide, PhD, Visiting Fellow, NIH/NIST Joint Sponsorship Program
- Kely L. Sheldon, MS, Predoctoral Intramural Research Training Award Fellow
In this Section, we study mitochondrial and bacterial membrane proteins that form large beta-barrel channels. The proteins are not only the gateways of metabolite exchange between different cellular compartments and cells, they are also recognized as multifunctional membrane receptors and components of many toxins, which are beginning to emerge as novel drug targets. Our main strategy is to reconstitute the proteins into planar lipid membranes in order to study them at the single-molecule level by high-resolution recording of ionic currents. Combining physical theory of intermolecular interactions with experiments on channel reconstitution, we seek to develop new approaches to treatment of various diseases, for which regulation of transport through ion channels plays the key role.
We have extensive experience with reconstitution of VDAC (Voltage-Dependent Anion Channel) from the outer membrane of mitochondria, alpha-Hemolysin (toxin from Staphylococcus aureus), translocation pores of Bacillus anthracis (PA63), Clostridium botulinum (C2IIa), and Clostridium perfringens (Ib) binary toxins, Epsilon toxin (from Clostridium perfringens), OmpF (general bacterial porin from Escherichia coli), LamB (sugar-specific bacterial porin from Escherichia coli), OprF (porin from Pseudomonas aeruginosa), Syringomycin E (lipopeptide toxin from Pseudomonas syringae), and the bacterial peptide TisB involved in persister cell biofilm formation. We also use Gramicidin A (linear pentadecapeptide from Bacillus brevis) as a molecular sensor of membrane mechanical properties. To study the channel-forming proteins under precisely controlled conditions, we first isolate them from the host organisms, purify them, and then reconstitute them into planar lipid bilayer membranes. Our goal is to elucidate the physical principles and molecular mechanisms that control metabolite flux under normal and pathological conditions. Specifically, we study channel interactions with cytosolic proteins, newly synthesized drugs, such as blockers of the translocation pores of bacterial toxins, and with the lipid membrane as modified by lipid composition and volatile anesthetics. By learning the physics, chemistry, and physiology of channel functioning, we strive to design new agents and strategies to effectively correct the aberrant interactions associated with diseases that compromise human well-being and healthy development.
Alpha-synuclein lipid-dependent membrane binding and translocation through beta-barrel channels
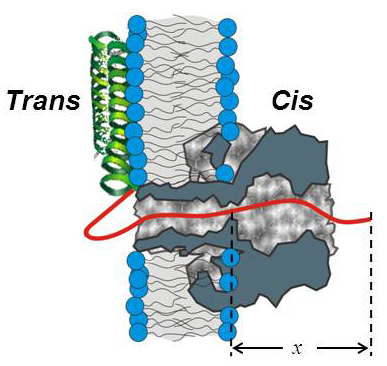
Figure 1. Cartoon of alpha-synuclein interaction with the alpha-hemolysin channel pore
The highly negatively charged C-terminal tail of the alpha-syn molecule (red solid line) enters the channel from the trans-side and goes past the channel constriction by length x (1).
Alpha-synuclein (alpha-syn), a neuronal water-soluble protein 140 amino-acids long, attracts great attention owing to its involvement in the etiology of Parkinson's disease (PD) and some other neurodegenerative dementias. Pathological hallmarks of PD include death of dopamine-secreting neurons in the substantia nigra and the presence of intracellular inclusions, or Lewy bodies, composed primarily of aggregated alpha-syn. Further evidence links gene multiplications and missense mutations in the alpha-syn gene to early-onset and familial PD. However, alpha-syn has not been directly implicated in cellular toxicity and neurodegeneration; moreover, its native cellular functions also remain a matter of debate. Gauging the interactions of alpha-syn with membranes and its pathways between and within cells is important for understanding its pathogenesis. This year, to address these questions, we used a robust beta-barrel channel, alpha-Hemolysin, reconstituted into planar lipid bilayers. Transient, approximately 95% blockage of the channel current by monomeric alpha-syn was observed when alpha-syn was added from the membrane side, where the shorter (stem) part of the channel is exposed, and the applied potential was lower on the side of alpha-syn addition. While the on-rate of alpha-syn binding to the channel strongly increased with the applied field, the off-rate displayed a turnover behavior. Statistical analysis suggests that, at voltages greater than 50 mV, a significant fraction of the alpha-syn molecules bound to the channel undergo subsequent translocation. The observed on-rate varied over 100-fold depending on the bilayer lipid composition. Removal of the last 25 amino acids from the highly negatively charged C-terminal of alpha-syn resulted in a significant lowering of the binding rates. Taken together, the results demonstrate that beta-barrel channels may serve as sensitive probes of alpha-syn interactions with membranes as well as model systems for studies of channel-assisted protein transport. The findings allow us to hypothesize that alpha-syn is also able to translocate through the human beta-barrel channel—the VDAC of the outer mitochondrial membrane—and therefore target complexes of the mitochondrial respiratory chain in the inner membrane. This may reveal the elusive physiological and pathophysiological roles for monomeric alpha-syn and thus reconcile and explain previous observations of the effects of this protein on mitochondrial bioenergetics.
VDAC regulation by tubulin
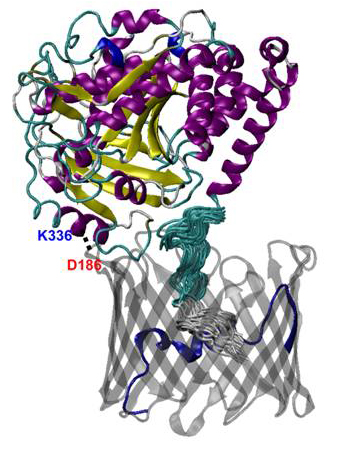
Figure 2. Molecular structure of the VDAC1−alpha-tubulin complex
The stable salt bridge between K336 of tubulin (shown in blue) and D186 of VDAC1 (shown in red) is illustrated by the dotted line (2).
Mitochondrial dysfunction is involved in the pathogenesis of numerous metabolic disorders of human development. We extended our studies on the VDAC of the outer mitochondrial membrane regulation by dimeric tubulin. Elucidating molecular interactions by which transport properties of this major channel of the outer mitochondrial membrane are modified by cytosolic proteins is important for understanding of mitochondrial functions that control cell survival and death. Using umbrella-sampling simulations of molecular dynamics (MD), we established the thermodynamic and kinetic components governing ATP transport across the VDAC1 channel. We found that there are several low-affinity binding sites for ATP along the translocation pathway and that the main barrier for ATP transport is located around the center of the channel and is formed predominantly by residues in the N-terminus. The binding affinity of ATP to an open channel was found to be in the millimolar to micromolar range. However, we showed that this weak binding raises the ATP translocation probability by about 10-fold compared with the VDAC pore in which attractive interactions had been artificially removed. We also applied methods of MD simulations to our recent finding that free dimeric tubulin induces a highly efficient, reversible blockage of VDAC reconstituted into planar lipid membranes and thus controls VDAC permeability for ATP/ADP and other mitochondrial respiratory substrates. Using the Rosetta protein-protein docking algorithm, we established a tentative structure of the VDAC−tubulin complex. An extensive set of equilibrium and nonequilibrium (under applied electric field) MD simulations were used to establish the conductance of the open and tubulin-blocked channel. We found that the presence of the unstructured C-terminal tail of tubulin in the VDAC pore lowers its conductance by more than 40% and switches its selectivity from anionic to cationic. The subsequent one-dimensional potential of mean force computation for the VDAC−tubulin complex shows that the state renders ATP transport virtually impossible. Several residues pivotal for tubulin binding to the channel were identified that help clarify the molecular details of VDAC-tubulin interaction and provide new insights into the mechanism of the control of mitochondria respiration by VDAC in health and disease.
Physical theory of transport
The so-called anomalous diffusion reported to take place at the surface of biological membranes and in the crowded environment of the cell has been attracting much attention recently. This year, we focused on creating analytical tools that would allow one to discriminate between bona fide anomalous diffusion and transient behavior in micro-heterogeneous environments. Free diffusion is a universal description of unbiased motion in a macro-homogeneous media and is widely used in the analysis of various problems in physics, chemistry, and biology. It is well known that diffusion in biological systems frequently occurs in heterogeneous environments, e.g., diffusion in the crowded cytoplasm, characterized by a wide range of scales. Therefore, it is not surprising that the data on the mean square displacements are frequently described by sub-linear dependences on time, whereas for free diffusion the mean square displacements should be proportional to the time of observation. Given that the sub-linear dependence is a fingerprint of anomalous subdiffusion, the question naturally arises as to how to distinguish (i) the phenomenon of anomalous subdiffusion from (ii) the transient behavior to the effective free diffusion regime (i.e., transient behavior that looks similar but is not subdiffusion per se). Three methods are most commonly used to characterize diffusion. One is to fit the time dependence of the mean square displacement to the function of time with the exponent as a free fitting parameter. Second is to integrate the dependence over time, find the area under the curve, and use it to obtain the exponent. Third is to differentiate the mean square displacement with respect to time and use the result for exponent determination. We showed that, when diffusion is anomalous, all three methods lead to the same value of the exponent; however, in the case of transient behavior, the three methods yield the time-dependent exponents, which, importantly, are different functions of time. In a separate study, we analyzed diffusion in a comb-like structure, formed by a main cylindrical tube with identical periodic dead ends. We developed a formalism that allows us to study the mean square displacement of a particle along the structure axis and demonstrated that transient behavior occurs naturally in such a system, which, within certain intervals of observation times, can be interpreted as anomalous.
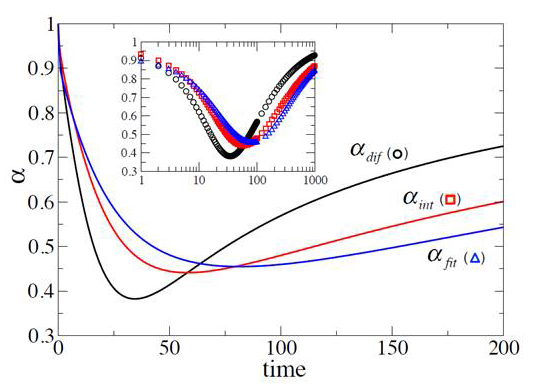
Figure 3. Discriminating between anomalous subdiffusion and transient behavior
Three exponents obtained from three different interpretations of the transient behavior of the mean square displacement as anomalous subdiffusion for a particle in the presence of periodically spaced permeable membranes (3).
Additional Funding
- NRC grant “Developing a bio-mimetic activating platform for the mitochondrial outer membrane” from NIH(NIBIB)/NIST Joint Sponsorship Program (2013-2015).
Publications
- Gurnev PA, Yap TL, Pfefferkorn CM, Rostovtseva TK, Berezhkovskii AM, Lee JC, Parsegian VA, Bezrukov SM. Alpha-synuclein lipid-dependent membrane binding and translocation through the alpha-hemolysin channel. Biophys J 2014;106:556-565.
- Noskov SY, Rostovtseva TK, Bezrukov SM. ATP transport through VDAC and VDAC/tubulin complex probed by equilibrium and non-equilibrium MD simulations. Biochemistry 2013;52:9246-9256.
- Berezhkovskii AM, Dagdug L, Bezrukov SM. Discriminating between anomalous diffusion and transient behavior in micro-heterogeneous environments. Biophys J 2014;106:L09-L11.
- Nestorovich EM, Bezrukov SM. Designing inhibitors of anthrax toxin. Expert Opin Drug Discov 2014;9:299-318.
- Teijido O, Rappaport SM, Chamberlin A, Noskov SY, Aguilella VM, Rostovtseva TK, Bezrukov SM. Acidification asymmetrically affects voltage-dependent anion channel implicating the involvement of salt bridges. J Biol Chem 2014;289:23670-23682.
Collaborators
- Vicente M. Aguilella, PhD, Universidad Jaume I, Castellón, Spain
- Alexander M. Berezhkovskii, PhD, Division of Computational Bioscience, CIT, NIH, Bethesda, MD
- Susan K. Buchanan, PhD, Laboratory of Molecular Biology, NIDDK, Bethesda, MD
- Leonid V. Chernomordik, PhD, Program in Physical Biology, NICHD, Bethesda, MD
- Leonardo Dagdug, PhD, Universidad Autónoma Metropolitana-Iztapalapa, Mexico City, Mexico
- Philip A. Gurnev, PhD, University of Massachusetts, Amherst, MA
- Jennifer C. Lee, PhD, Biochemistry and Biophysics Center, NHLBI, Bethesda
- John J. Lemasters, PhD, Medical University of South Carolina, Charleston, SC
- Ekaterina M. Nestorovich, PhD, The Catholic University of America, Washington, DC
- Sergei Y. Noskov, PhD, University of Calgary, Calgary, Canada
- Adrian Parsegian, PhD, University of Massachusetts Amherst, Amherst, MA
- Olga Protchenko, PhD, Liver Diseases Branch, NIDDK, Bethesda, MD
- Dan Sackett, PhD, Program in Physical Biology, NICHD, Bethesda, MD
- Gerhard Wagner, PhD, Harvard Medical School, Cambridge, MA
- Michael Weinrich, MD, National Center for Medical Rehabilitation Research, NICHD, Bethesda, MD
- David L. Worcester, PhD, National Institute of Standards and Technology, Gaithersburg, MD
- Joshua Zimmerberg, MD, PhD, Program in Physical Biology, NICHD, Bethesda, MD
Contact
For more information, email bezrukov@helix.nih.gov or visit smt.nichd.nih.gov.