You are here: Home > Section on Membrane Biology
Membrane Rearrangements in Macrophage Fusion and in Myoblast Fusion
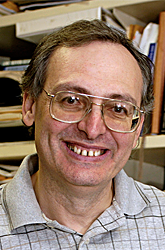
- Leonid V. Chernomordik, PhD, Head, Section on Membrane Biology
- Eugenia Leikina, DVM, Senior Research Assistant
- Kamram Melikov, PhD, Staff Scientist
- Elena Zaitseva, PhD, Research Fellow
- Santosh K. Verma, PhD, Visiting Fellow
- Berna Uygur, PhD, Postdoctoral Intramural Research Training Award Fellow
- Kwabena Yamoah, BS, Postbaccalaureate Fellow
Disparate membrane remodeling reactions are tightly controlled by protein machinery but are also dependent on the lipid composition of the membranes. Whereas each kind of protein has its own personality, membrane lipid bilayers have rather general properties manifested by their resistance to disruption and bending. Our long-term goal is to understand how proteins remodel membrane lipid bilayers in important cell biology processes. We expect that the analysis of the molecular mechanisms of important and diverse membrane rearrangements will clarify the generality of emerging mechanistic insights. Better understanding of fusion processes will bring about new ways of controlling them and lead to new strategies for quelling diseases involving cell invasion by enveloped viruses, intracellular trafficking, and intercellular fusion. Our general strategy is to combine in-depth analysis of the best-characterized fusion reactions with comparative analysis of diverse, less explored fusion reactions, which can reveal new kinds of fusion proteins and mechanisms. In recent studies, we focused on macrophage fusion in bone remodeling and on myoblast fusion in spinal muscular atrophy.
Dynamin-dependent stages of the synchronized macrophage fusion in osteoclast formation
Our bones are constantly remodeled by coordinated activity of bone-forming and bone-resorbing cells. Osteoclasts, multinucleated giant cells responsible for bone resorption in healthy animals and in metabolic, degenerative, and neoplastic bone disorders, are formed by fusion between the cells of the monocyte/macrophage hematopoietic lineage (“macrophages”). While the fusion process is essential for the bone-resorbing activity of osteoclasts in bone homeostasis, and, more generally, one of the most important examples of cell-to-cell fusion in development, tissue homeostasis, and the immune response, little is known about proteins that mediate the cell-fusion stage of osteoclastogenesis. One of the key challenges in exploring molecular mechanisms of this fusion process is to uncouple fusion from the complex multistep processes that prepare the cells for fusion and which take several days. Differentiation and migration that brings osteoclasts into pre-fusion contacts depend on many intracellular and extracellular proteins. However, finding that a specific protein activity is required for the formation of multinucleated cells does not necessarily implicate the particular protein in the cell-fusion stage. It is important to verify that such proteins and structures are involved in fusion rather than in some pre-fusion processes and, if they are, to identify the specific fusion stage. Cell-cell fusion starts with opening of nanometer-sized fusion pores, which connect the volumes of fusing cells. The pores can close or give rise to long-living membrane nanotubes connecting cells. In contrast, formation of multinucleated cells requires an expansion of the fusion pores. Early and late cell-cell fusion stages (formation of nascent membrane connections and their expansion) can be controlled by distinct protein machines, and syncytium formation can be inhibited downstream of fusion-pore opening.
To explore fusion between murine macrophage-like RAW cells and between macrophages generated from human monocytes, we recently uncoupled the fusion stage from pre-fusion processes using lysophosphatidylcholine (1). Lysophosphatidylcholine (LPC) reversibly blocks hemifusion, i.e., the merger of the contacting leaflets of two membranes at the onset of their fusion. Thus, an LPC block allowed us to accumulate ready-to-fuse macrophages and to observe their relatively synchronized fusion upon LPC removal. We concentrated cell-cell fusion events in osteoclast formation, which normally develop within 16h, to within 30–90 min. Thus, while osteoclastogenesis, generally, takes several days, our approach allowed us to focus on an hour, in which we observed robust fusion between the cells. To detect both completed cell-fusion events and fusion events stalled downstream of a local merger between cell membranes, we complemented the syncytium formation assay—the conventional way of quantifying osteoclast fusion—with an assay in which we detected early membrane connections as appearance of co-labeled cells after co-incubation of differently labeled fusion-committed macrophages.
In our recent studies, we have found that cell-cell fusion initiated by viral fusogens and myoblast fusion depend on dynamin. More recently, we explored the role of dynamin in osteoclast fusion. Suppression of dynamin 2 expression with siRNA resulted in the inhibition of formation of multinucleated osteoclasts. To test whether synchronized osteoclast fusion also depends on dynamin, we studied the effects of the dynamin GTP-ase inhibitors dynasore and MitMAB applied at the time of LPC removal. Both inhibitors suppressed syncytium formation but did not suppress membrane merger, suggesting that dynamin is involved in expansion of nascent membrane connections at the late stages of the cell fusion. While a paper describing this work was under review (2), Shin et al. (J Cell Biol 2014;207:73-89) also reported the importance of dynamin in osteoclast fusion.
While the specific mechanisms underlying dynamin-dependence of macrophage fusion remain to be clarified, our work emphasizes the conserved mechanistic motifs shared by cell-cell fusion processes in osteoclast formation, muscle development and regeneration and, possibly, by other cell-cell fusions. In addition to the conserved dependence of late fusion stages on dynamin, our finding that the hemifusion-inhibitor LPC reversibly blocks fusion of macrophages upstream of membrane merger indicates that macrophage fusion, as many other well-characterized fusion processes, proceeds via a fusion-through-hemifusion pathway. Future identification and characterization of the proteins that mediate the membrane merger stage of osteoclast formation, facilitated by our fusion synchronization approach, should elucidate the similarities and distinctions between fusion proteins that initiate diverse cell-cell fusions.
Myoblast fusion defects in spinal muscular atrophy
Understanding mechanisms that control myoblast fusion is important for developing new therapies to accelerate muscle regeneration and may bring useful insights into cancer cachexia, some myopathies, and muscular dystrophies. Our novel approaches and assays allowed us to isolate the event of merger of two plasma membranes from processes that prepare the cells for fusion and from processes that underlie the transition from early fusion connections to syncytium formation (1). We then focused on the potential role of myoblast fusion defects in spinal muscular atrophy (SMA), a hereditary disease that causes motor neuron degeneration and general muscle wasting. SMA, the most frequent inherited cause of infant mortality and the second most common fatal autosomal recessive disorder, affects 1 in 10,000 people, mostly children. The mechanisms by which mutations in the gene encoding survival motor neuron protein (SMN) cause progressive muscle atrophy remain elusive, and several studies have suggested a role for muscle, specifically myoblast fusion deficiency, in the pathophysiology of SMA. Research on cultured cells and animal models suggests that SMN deficiency in muscles causes a phenotype reminiscent of that observed in mouse models of muscular dystrophy, pointing to intrinsic muscle defects. Moreover, a recent study demonstrated that rescuing SMN in muscle alone improves the lifespan of SMA model mice, suggesting that skeletal muscles represent important targets for therapeutic intervention in SMA. SMA muscle has been reported to have smaller myotubes, suggesting a delay in myotube growth and maturation for SMN–deficient myoblasts and possible defects in myoblast fusion. We investigated the role of SMN in muscle development, using muscle cell lines and primary myoblasts, and confirmed earlier reports that SMN–deficient myoblasts fuse much less efficiently than wild-type cells (3). Importantly, the fusion defect was attributable to reduced SMN levels; transfecting SMN–deficient cells to express SMN rescued the fusion to the levels approaching those of wild-type myotubes. The local merger between myoblast plasma membranes and subsequent expansion of nascent fusion connections are two stages of myoblast fusion that are controlled by distinct protein machineries. To test whether SMN deficiency blocks myotube formation during differentiation downstream from membrane merger, we co-plated myoblasts labeled with different membrane probes. Merger of the membranes of differently labeled cells generates co-labeled cells. As expected, for wild-type myoblasts, we observed the appearance of multinucleated and co-labeled cells. In contrast, in the case of SMN–deficient myoblasts, we observed neither syncytium formation nor co-labeled cells. The finding indicates that the lack of SMN inhibits myotube formation upstream of even the earliest stages of myoblast fusion. Future work will show whether an enhancement of early stages of impaired myoblast may attenuate the fusion defect in SMN–deficient myoblasts and slow down muscle atrophy in SMA.
Additional Funding
- NIH's Intramural AIDS Targeted Antiviral Program (IATAP) 2013-2014
Publications
- Leikina E, Melikov K, Sanyal S, Verma SK, Eun B, Gebert C, Pfeifer K, Lizunov VA, Kozlov MM, Chernomordik LV. Extracellular annexins and dynamin are important for sequential steps in myoblast fusion. J Cell Biol 2013;200:109-123.
- Verma S, Leikina E, Melikov K, Chernomordik LV. Late stages of the synchronized macrophage fusion in osteoclast formation depend on dynamin. Biochem J 2014;E-pub ahead of print.
- Bricceno KV, Martinez T, Leikina E, Duguez S, Partridge TA, Chernomordik LV, Fischbeck KH, Sumner CJ, Burnett BG. Survival motor neuron protein deficiency impairs myotube formation by altering myogenic gene expression and focal adhesion dynamics. Hum Mol Genet 2014;23:4745-4757.
- Kozlov MM, Campelo F, Liska N, Chernomordik LV, Marrink SJ, McMahon HT. Mechanisms shaping cell membranes. Curr Opin Cell Biol 2014;29C:53-60.
Collaborators
- Katherine Bricceno, PhD, Neurogenetics Branch, NINDS, Bethesda, MD
- Barrington G. Burnett, PhD, Uniformed Services University of the Health Sciences, Bethesda, MD
- Kenneth H. Fischbeck, PhD, Neurogenetics Branch, NINDS, Bethesda, MD
- Claudia M. Gebert, PhD, Program in Genomics of Differentiation, NICHD, Bethesda, MD
- Jyoti Jaiswal, PhD, George Washington University School of Medicine and Health Sciences, Washington, DC
- Michael M. Kozlov, PhD, DHabil, Sackler Faculty of Medicine, Tel Aviv University, Tel Aviv, Israel
- Karl Pfeifer, PhD, Program in Genomics of Differentiation, NICHD, Bethesda, MD
- Benjamin Podbilewicz, PhD, Technion-Israel Institute of Technology, Haifa, Israel
Contact
For more information, email chernoml@mail.nih.gov.