You are here: Home > Section on Cell Biophysics
Cell Biophysics
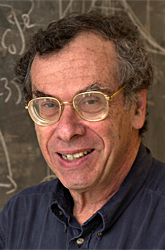
- Ralph Nossal, PhD, Head, Section on Cell Biophysics
- Dan Sackett, PhD, Staff Scientist
- Anand Banerjee, PhD, Visiting Fellow
- Felipe Montecinos, PhD, Visiting Fellow
- Alex Szatmary, PhD, Postdoctoral Fellow
- Hacène Boukari, PhD, Guest Researcher
- Norman Gershfeld, PhD, Guest Researcher
- Matthew Mirigian, BA, Guest Researcher
Our investigations center on how cell behavior is linked to underlying physical mechanisms, for which we develop and apply methodologies based on mathematical and physical principles. Projects currently include: (i) elaborating a physical model to explain the stochastic nature of coated vesicle biogenesis during receptor-mediated endocytosis, focusing on how nanoparticle uptake relies on mesoscopic cell mechanics; (ii) understanding the nature and consequences of temperature-linked phase transitions in biological cell membranes, particularly those occurring near the temperature at which the cells grow and are maintained; (iii) exploring how substrate mechanical properties and diffusible chemotaxins affect the response of eukaryotic cells to changes in their environment; and (iv) understanding how certain small molecules interact with microtubules and thereby act as antimitotic agents, and how microtubule arrays function in mitosis to produce accurate segregation of chromosomes. We have a particular interest in the ways cellular activities are coordinated in space and time.
Complex systems biophysics
We have been employing physical and mathematical methods to understand the biophysics of complex cellular processes. A major focus has been the biogenesis of coated vesicles involved in clathrin-mediated endocytosis (CME) and other intracellular transport processes. CME is the principal pathway for the regulation of receptors and the internalization of certain nutrients and signaling molecules at the plasma membrane of eukaryotic cells. The early stage of receptor-mediated endocytosis involves the formation of transient structures known as clathrin-coated pits (CCPs) which, depending on the detailed energetics of protein binding and associated membrane transformations, either mature into clathrin-coated vesicles (CCVs) or regress and vanish from the cell surface. The former are referred to as “productive” CCPs and the latter as "abortive" CCPs. We previously developed a simple physical model for CCP dynamics and carried out Monte Carlo simulations to investigate the time development of CCP size, explain the origin of abortive pits, and reproduce features of their lifetime distribution. By fitting our results to experimental data, we estimated values of the free energy changes involved in formation of the protein coat that surrounds a CCV, and we showed how the binding of cargo might modify the coat parameters and thereby facilitate CCV formation. We also derived analytical expressions for various characteristic quantities, including the lifetime distribution and the distribution of maximum sizes of abortive pits, the probability that, once started, a CCP is productive, and the mean time for the growth of a CCP to the point where it is released from the plasma membrane. Using these expressions, we then calculated the mean time between the binding of a nanoparticle (NP) to a cell surface and its internalization as a result of CME. The approach enabled us to show that NP internalization occurs most efficiently when NP size is commensurate with the natural curvature of the clathrin-containing protein coat of the vesicle, but that uptake is not significantly different for sizes falling within minimum and maximum bounds determined by energy parameters such as the free energy associated with coat formation and the bending rigidities of the coat and membrane components. We showed that these observations are consequences of the kinetics of protein coat formation during CCP production.
We have also been developing predictive models to illuminate other aspects of receptor-mediated endocytosis (RME), of which CME is the best characterized. For example, RNAi depletion experiments indicate that a well-defined threshold value of intracellular clathrin must be exceeded before CCVs are produced and that empty clathrin cages are rarely seen in the cytoplasm. To understand these observations, we used a thermodynamic model to investigate the conditions under which clathrin triskelions form polyhedral baskets. The analysis, which is similar to classical methods used to study micelle formation, relates clathrin-basket energetics to system parameters that are linked to triskelial rigidity, the natural curvature of an isolated triskelion, and interactions between triskelial legs in the assembled polyhedra. The theory predicts that a minimal ("critical") concentration must be surpassed in order for basket polymerization to occur and indicates how this quantity and the amount of polymerized material depend on the chosen parameters. In particular, we obtained a general mathematical expression that relates the critical concentration to the free energy change that occurs when baskets of maximal prevalence assemble. Using explicit expressions for this quantity, we confirmed that the critical concentration is lowered by factors that increase basket stability. Various cell processes compete for clathrin, including interactions with microtubule-binding proteins during mitosis and processes occurring at endosomes and the Golgi, suggesting that critical concentration biophysics may be an important factor in the global control of cell activities involving clathrin.
Our research also involves analyzing and understanding the significance of temperature-dependent lipid phase transitions associated with biological processes. Previous work in our laboratories indicated that whole lipid extracts from prokaryotes exhibit a phase transition at a temperature T* corresponding to the growth temperatures of the organisms; lipid extracts obtained from neural tissues gave similar results. We recently examined previously published data concerning the temperature dependence of the neural responses of intact tissues from frog, squid, and rat. The temperature dependencies of the resting potential and action potential of frog and squid exhibit behaviors that strongly suggest the onset of a membrane state change when T>Tg, where Tg is the gestation temperature of a particular organism. Anomalies in the flow of current in the rat ganglion lead to a similar conclusion. Based on approximations to the classic Hodgkin-Huxley equations, analysis of axonal response indicates that observed changes in the resting potential and peak of the action potential reflect chemo-mechanical coupling between voltage-switchable ion channels and temperature-linked, lipid phases of the plasma membrane. The behavior of the resting potential and action potential when T>Tg can be rationalized by postulating the emergence of a different lipid state that alters channel properties. The change in neural properties with increasing temperature for these animals has an activation energy of approximately 26 kcal/mole. Short exposure to temperatures above Tg alters the stability of the channels, whereas elevating the temperature for longer periods appears to result in irreversible degradation of the tissue.
Tubulin polymers and cytoskeletal organization
We continued our study of the properties of microtubules (MT), and of drugs that alter these properties, and of the biology of MT arrays, such as the mitotic spindle. Our recent work identified the binding site for a new microtubule-stabilizing drug. In the course of this work, we needed to revisit the biophysical methods used for monitoring drug effects on the polymerization of MT in vitro. In addition, we addressed some mitosis-specific proteins that affect the fidelity of mitosis and which may be important in generating chromosome defects seen in cancer.
Microtubule-targeting drugs, and other chemotherapy agents, cause an increase in cellular reactive oxygen species (ROS) as direct or ancillary effects of their intracellular action. Some such increases are known to be attributable to effects on mitochondria while others to unknown mechanisms. Mitochondrial ROS production may occur after exposure of cells to microtubule-directed drugs, owing to changes in tubulin interaction with the mitochondrial outer membrane. We found that oxidation of tubulin carboxy-terminal tail peptides alters the mitochondrial interaction in a way that significantly increases the residence time for tubulin once bound to the membrane. The binding stops metabolite traffic into and out of the mitochondria. While the work is still incomplete, it is possible that this is a significant source of anti-microtubule drug-induced oxidative damage in the cell.
We examined oxidative damage in cells by developing a new method to detect the oxidation in live cells. We built on our previous use of “click-chemistry” to design a cell-permeable fluorophore that reacts with oxidized molecules. The molecules often contain carbonyl groups as a result of oxidation, and our click chemistry approach pairs fluorophores with hydrazine moieties to react spontaneously and specifically with the carbonyl groups. The result is the covalent attachment of fluorophores to the sites of oxidation in the cell, which can then be visualized in the fluorescence microscope or identified biochemically following cell lysis and chromatography. The details of the protocol are not yet set, and we are attempting to develop the assay with fluorophores with longer excitation wavelengths than the current UV–requiring molecule.
Polymerization of MT from purified tubulin, the major structural protein in MT, is an accepted model for quantitatively evaluating the effects of established MT–targeting drugs, as well as for screening collections of compounds for new MT–targeting agents. The most common method is to monitor the increase in light scattering produced by inducing a solution of tubulin to polymerize into MT. This is usually measured with a simple spectrophotometer, using long-wave UV light (typically 350 nm). While an increase provides an unambiguous signal of the formation of larger structures in the solution, the mere fact of increased light scattering does not mean that the larger structures are MT; random aggregation would also produce an increase in signal. We demonstrated how a slightly more careful approach to this method than is commonly used will yield much more information, including a rough check that the polymers produced are MT. We also combined this with fluorescence methods for monitoring polymerization that are somewhat more resistant to the effects of aggregation.
MT–targeting drugs induce arrest of mitosis in rapidly dividing cells but also alter MT function during the 95% or more of the time that a cell is not in mitosis. The latter functions are likely the reason that such drugs are effective chemotherapy agents against many human cancers. Even if mitosis is not the main target of these drugs in tumors, as seems to be the case, it is nonetheless clear that cancer cells accumulate many chromosomal defects that originate in faulty mitosis. One such common defect is aneuploidy, in which cells have abnormal numbers of chromosomes, likely a result of problems in the functional fidelity of the mitotic spindle. We previously studied the protein CKAP2, which serves to enhance mitotic fidelity and prevent aneuploidy. We have since been studying a new assay for molecules that either enhance or reduce mitotic fidelity. The assay will be tested with CKAP2 by engineering cells with reduced or enhanced CKAP2 and other proteins that might alter mitotic fidelity. Currently, we are using it to test and compare various drugs of interest, including microtubule-targeting chemotherapy agents. The assay uses a cell line that contains a Human Artificial Chromosome (HAC) that carries a gene for GFP (green fluorescent protein). The HAC is maintained in the cells by the action of a drug that selects for cells that retain the HAC and a gene that encodes a detoxifying protein. For the assay, the maintenance drug is removed and the test drugs are added. As cells divide, the HAC is lost from cells with compromised mitotic fidelity. The rate of loss is measured by flow-cytometric detection of GFP, which allows comparison of various drugs. We finished comparing the major microtubule-targeting drugs and several other chemotherapy agents and environmental toxins. Thus far, the microtubule-targeting drugs are the most active of any class of drugs, but the comparisons are not yet complete. The protocol will allow quantitation of an important aspect of the action of many drugs used clinically, as well as many man-made compounds or naturally occurring environmental toxins.
Biophysical methods and models
We continue to develop novel physics-based methodologies for investigating complex biological materials and processes. We recently carried out several studies related to the response of eukaryotic cells to environmental cues. It is becoming increasingly clear that the cell microenvironment, including the surrounding extracellular matrix, profoundly affects cell fate. This is especially true for solid tumors, in which matrix stiffness, for example, is believed to be an important factor in tumorigenesis. Given that matrix stiffness affects cell fate, it may also be important in drug resistance. Consequently, we designed and built a multiwell, “high-throughput,” polyacrylamide (PA) gel–based stiffness assay. The gels were coated with collagen in order to facilitate cell attachment and proliferation, and the polyacrylamide substrates were fabricated with stiffness values ranging from 0.3 to 300 kPa. The high-throughput format facilitates obtaining dose-response curves through simultaneous testing of several parameters. Additionally, the assay is an improvement on other techniques in terms of preparation time, robustness, and cost. We thereby examined the effect of stiffness on cultured cell responsiveness to cytotoxic drugs. In particular, we demonstrated the utility of the assay by testing multiple cancer cell lines and their susceptibility to paclitaxel, a microtubule-targeting agent.
A second study was directed at improving assays for eukaryotic cell chemotaxis, specifically, for quantitative assessment of the chemotactic response of polymorphonuclear (PMN) leukocytes and other amoeboid cells. Migration of cells along gradients of effector molecules, i.e., chemotaxis, is necessary during immune response and is involved in tissue development and cancer metastasis. The experimental assessment of chemotaxis is therefore of high interest. The agarose spot assay is a simple tissue-culture system used to detect directed cell movement induced by gradients of diverse materials, such as peptides released from the sites of bacterial infection and macromolecules that serve as growth factors. Direction sensing requires that gradients be appropriately steep, and it is necessary to know how the chemical gradients developed in the assay change over time so that proper conditions for measuring chemotaxis prevail. We used numerical solution of the Diffusion Equation to determine the chemoattractant gradient produced in the assay. Our analysis shows that, for the usual spot size, the lifetime of the assay is optimized if the chemoattractant concentration in the spot is initially 30 times the dissociation constant of the chemoattractant-receptor bond. The result holds regardless of the properties of the chemoattractant. With this initial concentration, the chemoattractant gradient falls to the minimum threshold for directional sensing at the same time as the concentration drops to the optimal level for detecting gradient direction. If a higher initial chemoattractant concentration is used, the useful lifetime of the assay is likely to be shortened because receptor saturation may decrease the cells’ sensitivity to the gradient, whereas lower initial concentrations would result in too little chemoattractant for the cells to detect. Moreover, chemoattractants with higher diffusion coefficients would sustain gradients for less time. Based on previous measurements of the diffusion coefficients of the chemoattractants EGF and CXCL12, we estimate that the assay will produce gradients that cells can sense for a duration of 10 h for EGF and 5 h for CXCL12. These and other results of our analysis provide guidelines for applying this inexpensive and easily implemented method. Related assays also are being analyzed, in conjunction with work directed at gaining a better understanding of the mechanism of cell adhesion and how it affects chemotaxis and other aspects of amoeboid locomotion.
In support of these and related studies, we continued our development of methods based on fluorescence correlation spectroscopy (FCS) that can be used to study the diffusion of nanoscopic fluorescent biomacromolecules within polymer solutions and gels. Specifically, we examined the movement of two “nanoprobes,” rhodamine R6G and TAMRA, in polyvinyl-alcohol (PVA) suspensions. We used PVA as a model system because the polymer has many attractive practical properties: it is water-soluble, neutral, optically transparent (both in solution or gel), and stable. Furthermore, many of its structural properties have been characterized by complementary techniques such as small-angle neutron scattering. To perform the FCS measurements, we designed an optical chamber to measure in situ changes of the diffusion of fluorescent nanoprobes while the PVA solutions and gels were dehydrating slowly by evaporation of water through a porous membrane (3500 Da porosity). With this custom-made, microscope-based FCS setup, we were able to monitor changes in fluorescence with time. We found, for example, that the nanoprobes slowed down as the pH was lowered, presumably owing to interactions between the probes and the PVA. Raising PVA concentration and crosslink density also induced slowing down. Dehydration induced a systematic reduction in the diffusion of TAMRA in both solutions and gels; however, the changes in dehydrating gels are greater than in the corresponding PVA solutions. The results demonstrate that FCS is a powerful, relatively non-invasive technique to probe in situ interactions (hydrodynamics or others) of nanoprobes in polymer systems. We plan to use this and related techniques to study the diffusion of chemotaxins through agarose and other polymer matrices.
Publications
- Muthukumar M, Nossal, R. Micelle-like model for the polymerization of clathrin baskets. J Chem Phys 2013;139:121928.
- Mirigian M, Mukherjee K, Bane SL, Sackett DL. Measurement of in vitro microtubule polymerization by turbidity and fluorescence. Methods Cell Biol 2013;115:215-229.
- Case CM, Sackett DL, Wangsa D, Karpova T, McNally JG, Ried T, Camps J. CKAP2 ensues chromosomal stability by maintaining the integrity of microtubules. PLoS One 2013;8:e64575.
- Zustiak SP, Nossal R, Sackett DL. Multiwell stiffness assay for the study of cell responsiveness to cytotoxic drugs. Biotech Bioeng 2014;111:396-403.
- Szatmary AC, Stuelten CH, Nossal R. Improving the design of the agarose spot assay for eukaryotic cell chemotaxis. RSC Advances 2014;in press.
Collaborators
- Susan Bane, PhD, Binghamton University, Binghamton, NY
- Alexander Berezhkovskii, PhD, Division of Computational Bioscience, CIT, NIH, Bethesda, MD
- Hacène Boukari, PhD, Delaware State University, Dover, DE
- Tito Fojo, MD, PhD, Medical Oncology Branch, NCI, Bethesda, MD
- Amir Gandjbakhche, PhD, Program in Pediatric Imaging and Tissue Sciences, NICHD, Bethesda, MD
- Svetlana Glushakova, MD, PhD, Program in Physical Biology, NICHD, Bethesda, MD
- Ferenc Horkay, PhD, Program in Pediatric Imaging and Tissue Sciences, NICHD, Bethesda, MD
- Albert J. Jin, PhD, Laboratory of Cellular Imaging and Macromolecular Biophysics, NIBIB, Bethesda, MD
- Eileen Lafer, PhD, University of Texas Health Science Center, San Antonio, TX
- Vladimir Larionov, PhD, Developmental Therapeutics Branch, NCI, Bethesda, MD
- Philip McQueen, PhD, Division of Computational Bioscience, CIT, NIH, Bethesda, MD
- Muraggapan Muthukumar, PhD, University of Massachusetts, Amherst, MA
- David Sept, PhD, University of Michigan, Ann Arbor, MI
- Richard Taylor, PhD, Notre Dame University, Notre Dame, IN
- Christina Stuelten, MD, PhD, Laboratory of Cellular and Molecular Biology, NCI, Bethesda, MD
- Al Yergey, PhD, Mass Spectrometry Core Facility, NICHD, Bethesda, MD
- Joshua Zimmerberg, MD, PhD, Program in Physical Biology, NICHD, Bethesda, MD
- Silviya Zustiak, PhD, Saint Louis University, St. Louis, MO
Contact
For more information, email nossalr@mail.nih.gov.