You are here: Home > Section on Cellular and Synaptic Physiology
Hippocampal Interneurons and Their Role in the Control of Network Excitability

- Chris J. McBain, PhD, Head, Section on Cellular and Synaptic Physiology
- Kenneth Pelkey, PhD, Staff Scientist
- Ramesh Chittajulla, PhD, Senior Research Fellow
- Christian Cea del Rio, BS, Visiting Fellow
- Jose Matta, PhD, Visiting Fellow
- Christine Torborg, PhD, Visiting Fellow
- Ludovic Tricoire, PhD, Visiting Fellow
- Sean Barron, PhD, Postdoctoral Fellow
- Brian Erkkila, PhD, Visiting Fellow
- Brian Jefferies, BS, Biologist
- Xiaoqing Yuan, MSc, Biologist
- Elizabeth Mayne, BSc, Graduate Student
- Madhav Sukumaran, BSc, Graduate Student
Cortical and hippocampal local circuit GABAergic inhibitory interneurons are "tailor-made" to control Na+- and Ca2+-dependent action potential generation, to regulate synaptic transmission and plasticity, and to pace large-scale synchronous oscillatory activity. The axons of this diverse cell population make local, short-range projections (although some subpopulations project their axons over considerable distances) and release the inhibitory neurotransmitter gamma-aminobutyric acid (GABA) onto a variety of targets. A mounting appreciation of the roles played by interneurons in several mental health conditions such as epilepsy, stroke, Alzheimer's disease and schizophrenia have placed this important cell type center stage in cortical circuit research. Our main objective is to understand how both ionic and synaptic mechanisms regulate the activity of local circuit GABAergic interneurons and principal neurons at the level of small well-defined networks. To this end, we use a variety of electrophysiological, immunohistochemical, molecular, and genetic approaches in both wild-type and transgenic animals. Over the past five years, we have continued our study of the differential mechanisms of glutamatergic and GABAergic synaptic transmission and plasticity within the hippocampal formation and the modulation of voltage- and ligand-gated channels expressed in inhibitory neurons. We have also incorporated genetic approaches to unravel the embryogenesis and development of hippocampal interneurons and the circuits in which they are embedded.
Narp regulates homeostatic scaling of excitatory synapses on parvalbumin interneurons.
To compensate for prolonged changes in network activity, homeostatic synaptic scaling alters the strength of synapses; it involves both excitatory and inhibitory neurons. The immediate-early gene Narp (neuronal activity–regulated pentraxin) encodes a secreted synaptic protein that can bind to and induce clustering of AMPA receptors (AMPARs). We found that Narp prominently accumulated at excitatory synapses on parvalbumin-expressing interneurons (PV-INs). Increasing network activity resulted in a homeostatic increase of excitatory synaptic strength onto PV-INs that enhanced inhibitory drive—a response that was absent from neurons cultured from Narp−/− mice. Activity-dependent changes in the strength of excitatory inputs on PV-INs in acute hippocampal slices were also dependent on Narp, and Narp−/− mice had elevated sensitivity to kindling-induced seizures. We propose that Narp recruits AMPARs at excitatory synapses onto PV-INs to rebalance network excitation/inhibition dynamics following episodes of increased circuit activity.
Control of CA3 output by feedforward inhibition despite developmental changes in the excitation-inhibition balance
In the somatosensory cortex, the relative balance of excitation and inhibition determines the effectiveness of feedforward inhibition in enforcing the temporal fidelity of action potentials (APs). Within the CA3 region of the hippocampus, glutamatergic mossy fiber (MF) synapses onto CA3 pyramidal cells (PCs) provide strong monosynaptic excitation that exhibits prominent facilitation during repetitive activity. We demonstrated in the juvenile CA3 that MF-driven polysynaptic inhibitory postsynaptic currents (IPSCs) facilitate to maintain a fixed ratio of excitatory postsynaptic current (EPSC) to IPSC during short-term plasticity. In contrast, in young adult mice, the MF–driven polysynaptic inhibitory input can facilitate or depress in response to short trains of activity. Transgenic mice lacking the feedback inhibitory loop continue to exhibit both facilitating and depressing polysynaptic IPSCs, indicating that this robust inhibition may not be attributed to the secondary engagement of feedback inhibition. Surprisingly, eliminating MF–driven inhibition onto CA3 pyramidal cells by blockade of GABA A receptors did not lead to a loss of temporal precision of the first AP observed after a stimulus but, in many cases, triggered a long, excitatory plateau potential capable of triggering repetitive AP firing. The observations indicate that, unlike the case in other regions of the brain, the temporal precision of single MF–driven APs is dictated primarily by the kinetics of MF EPSPs, not by feedforward inhibition. Instead, feedforward inhibition provides robust regulation of CA3 PC excitability across development to prevent excessive depolarization by the monosynaptic EPSP and multiple AP firings.
M3 muscarinic acetylcholine receptor expression confers differential cholinergic modulation to neurochemically distinct hippocampal basket cell subtypes.
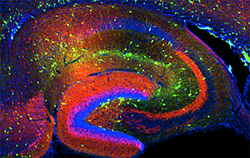
Click image to enlarge.
Figure 1. Interneurons in the GAD-65 GFP mouse
Numerous subpopulations are present within a hippocampal section derived from a GAD-65 GFP transgenic mouse.
Cholinergic neuromodulation of hippocampal circuitry promotes network oscillations and facilitates learning and memory through cellular actions on both excitatory and inhibitory circuits. Despite widespread recognition that neurochemical content discriminates between functionally distinct interneuron populations, there has been no systematic examination of whether neurochemically distinct interneuron classes undergo differential cholinergic neuromodulation in the hippocampus. Earlier, we described a stereotypic response of somatostatin-positive oriens lacunosum moleculare (OLM) cells to cholinergic receptor activation. In these cells, activation of M1 and M3 muscarinic receptors triggered an acceleration of AP firing that was accompanied by a conversion of the spike after-hyperpolarization to an after-depolarization. Building on this stereotypic response in an anatomically well-defined population of interneurons, Christian Cea-del Rio, Josh Lawrence, and Ludovic Tricoire then explored whether two classes of identifiable basket cell (BCs) similarly expressed stereotypic responses to muscarinic receptor activation. Using GFP transgenic mice that enable the visualization of perisomatically targeting parvalbumin-positive (PV+) or cholecystokinin-positive (CCK+) BCs, we tested the hypothesis that neurochemically distinct interneuron populations are differentially engaged by muscarinic acetylcholine receptor (mAChR) activation. Endogenous cholinergic fiber activation revealed that CCK BCs were more sensitive than PV BCs to synaptic release of ACh. Furthermore, in response to depolarizing current steps, mAChR activation of PV BCs and CCK BCs elicited distinct cholinergic response profiles, differing in mAChR–induced changes in AP waveform, firing frequency, and intrinsic excitability. In contrast to PV BCs, CCK BCs exhibited an mAChR–induced afterdepolarization (mADP) that was frequency- and activity-dependent. Pharmacological, molecular, and loss-of-function data all pointed to the presence of M3 mAChRs in distinguishing CCK BCs from PV BCs. Firing frequency of CCK BCs was controlled through M3 mAChRs, but PV BC excitability was altered solely through M1 mAChRs. Finally, upon mAChR activation, glutamatergic transmission enhanced cellular excitability preferentially in CCK BCs but not in PV BCs. The findings demonstrate that cell type–specific cholinergic specializations are present on neurochemically distinct interneuron subtypes in the hippocampus, revealing an organizing principle that cholinergic neuromodulation depends critically on neurochemical identity.
In a follow-up study, we observed that cholinergic modulation amplifies the intrinsic oscillatory properties of CA1 hippocampal CCK–positive interneurons. We showed that two types of CCK–positive inhibitory interneurons, the so-called basket cells and Schaffer collateral–associated cells, which separately innervate the cell body and dendritic regions of principal cells, are modulated similarly by cholinergic receptor activation. In both cell types, activation of their muscarinic receptors triggers a general increase of excitability and intrinsic oscillatory activity and a more efficient engagement to slow network oscillations. Knowledge of how cholinergic neuromodulation acts on neurochemically identical but morphologically distinct inhibitory interneurons will allow us to understand the role played by this important neuromodulator during hippocampal-dependent tasks in vivo.
The spatiotemporal origins of hippocampal interneuron diversity
Although vastly outnumbered, inhibitory interneurons critically pace and synchronize excitatory principal cell populations to coordinate cortical information processing. Precision in this control relies on a remarkable diversity of interneurons primarily determined during embryogenesis by genetic restriction of neuronal potential at the progenitor stage. Like their neocortical counterparts, hippocampal interneurons arise from medial and caudal ganglionic eminence (MGE and CGE) precursors. However, while studies of the early specification of neocortical interneurons are rapidly advancing, similar lineage analyses of hippocampal interneurons have lagged.
From our perspective, it became increasingly apparent that a "hippocampocentric" investigation of interneuron lineage analysis was needed because several hippocampal interneuron subtypes remain poorly represented in the neocortical literature. Thus, Ludovic Tricoire, Ken Pelkey, and Brian Erkkila investigated the spatiotemporal origins of hippocampal interneurons by using transgenic mice that specifically reported medial ganglionic eminence (MGE)– and caudal ganglionic eminence (CGE)–derived interneurons either constitutively or inducibly. We found that hippocampal interneurons are produced in two neurogenic waves between E9–E12 and E12–E16 from MGE and CGE, respectively. The cells migrate through the marginal zone and subventricular zone to populate the stratum lacunosum moleculare before they reach their final destination within the hippocampus proper. Migration from the MGE and CGE into the hippocampus takes varying amounts of time, with cells born at later embryonic stages taking less time despite the increased dimensions of the migratory path length. In the mature hippocampus, CGE–derived interneurons primarily localize to superficial layers in strata lacunosum moleculare and deep radiatum while MGE–derived interneurons readily populate all layers with preference for strata pyramidale and oriens. Combined molecular, anatomical, and electrophysiological interrogation of MGE/CGE–derived interneurons revealed that the MGE produces parvalbumin-, somatostatin-, and nitric oxide synthase–expressing interneurons, including fast-spiking basket, bistratified, axo-axonic, OLM, neurogliaform, and Ivy cells. In contrast, CGE–derived interneurons contain cholecystokinin, calretinin, vasoactive intestinal peptide, and reelin, including non–fast-spiking basket, Schaffer collateral–associated, mossy fiber–associated, trilaminar, and additional neurogliaform cells. Our findings provide a basic blueprint of the developmental origins of hippocampal interneuron diversity.
Common origins of nitric oxide synthase–expressing hippocampal Ivy and neurogliaform cells
GABAergic interneurons critically regulate cortical computation through exquisite spatiotemporal control over excitatory networks. Precision of this inhibitory control requires a remarkable diversity within interneuron populations that is largely specified during embryogenesis. Although interneurons expressing the neuronal isoform of nitric oxide synthase (nNOS) constitute the largest hippocampal interneuron cohort, their origin and specification remain unknown. Thus, as neurogliaform cells (NGC) and Ivy cells (IvC) represent the main nNOS interneurons, we investigated their developmental origins. Although considered distinct interneuron subtypes, NGCs and IvCs exhibited similar neurochemical and electrophysiological signatures, including NPY (neuropeptide Y) expression and late spiking. Moreover, lineage analyses, including loss-of-function experiments and inducible fate-mapping, indicated that nNOS IvCs and NGCs are both derived from MGE progenitors under the control of the transcription factor Nkx2-1. Surprisingly, a subset of NGCs lacking nNOS arises from CGE progenitors. Thus, while nNOS NGCs and IvCs arise from MGE progenitors, a CGE origin distinguishes a discrete population of nNOS NGCs.
Neto1 is an auxiliary subunit of native synaptic kainate receptors.
Although auxiliary proteins have been identified for AMPA and NMDA receptor complexes, little is known about kainate receptor (KAR) complex proteins. In collaboration with Mike Salter's and Rod McInnis' laboratories, Ken Pelkey showed that Neto1 is an auxiliary subunit for endogenous synaptic KARs. We found that Neto1 and KARs co-immunoprecipitated from brain lysates, from postsynaptic densities (PSDs) and, in a manner dependent on Neto1 CUB domains, when co-expressed in heterologous cells. In Neto1-null mice, we observed about a 50% reduction in the abundance of GluK2-KARs in hippocampal PSDs. Neto1 strongly localized to CA3 stratum lucidum, and loss of Neto1 resulted in a selective deficit in KAR–mediated neurotransmission at MF-CA3 synapses. KAR–mediated EPSCs in Neto1-null mice had lower amplitudes and decayed more rapidly than did those in wild-type mice. In contrast, the loss of Neto2, which also localizes to stratum lucidum and interacts with KARs, had no effect on KAR synaptic abundance or MF–CA3 transmission. Indeed, MF–CA3 KAR deficits in Neto1/Neto2 double-null mutant mice were indistinguishable from Neto1 single-null mice. Thus, our findings establish Neto1 as an auxiliary protein required for synaptic function of KARs. The ability of Neto1 to regulate both NMDA receptors and KARs reveals a unique dual role in controlling synaptic transmission by serving as an auxiliary protein for these two classes of ionotropic glutamate receptors in a synapse-specific fashion.
Additional Funding
- Dr. Torborg was the recipient of a Pharmacology Research Associate (PRAT) Fellowship.
- Dr. Matta was the recipient of a Pharmacology Research Associate (PRAT) Fellowship.
Publications
- Tricoire L, Pelkey KA, Daw MI, Sousa VH, Miyoshi G, Jeffries B, Cauli B, Fishell G, McBain CJ. Common origins of hippocampal Ivy and nitric oxide synthase expressing neurogliaform cells. J Neurosci 2010;30:2165-2176.
- Cea-del Rio CA, Lawrence JJ, Tricoire L, Erdelyi F, Szabo G, McBain CJ. M3 muscarinic acetylcholine receptor expression confers differential cholinergic modulation to neurochemically distinct hippocampal basket cell subtypes. J Neurosci 2010;30:6011-6024.
- Torborg CL, Nakashiba T, Tonegawa S, McBain CJ. Control of CA3 output by feedforward inhibition despite developmental changes in the excitation-inhibition balance. J Neurosci 2010;30:15628-15637.
- Tang M, Pelkey KA, Ng D, Ivakine E, McBain CJ, Salter MW, McInnes RR. Neto1 is an auxiliary subunit of native synaptic kainate receptors. J Neurosci 2011;31:10009-10018.
- Tricoire L, Pelkey KA, Erkkila BE, Jeffries BG, Yuan XQ, McBain CJ. A blueprint for the spatiotemporal origins of hippocampal interneuron diversity. J Neurosci 2011;31:10948-10970.
Collaborators
- Andres Buonanno, PhD, Program in Developmental Neuroscience, NICHD, Bethesda, MD
- Bruno Cauli, PhD, Université Pierre et Marie Curie, Paris, France
- Gordon Fishell, PhD, New York University, New York, NY
- Roderick McInnes, PhD, Lady Davis Research Institute, McGill University, Toronto, Canada
- Michael Salter, PhD, Centre for the Study of Pain, Hospital for Sick Children, Toronto, Canada
- Paul Worley, PhD, The Johns Hopkins University, Baltimore, MD
Contact
For more information, email mcbainc@mail.nih.gov or visit neuroscience.nih.gov/Faculty/Profile/chris-mcbain.aspx.