You are here: Home > Section on Sensory Coding and Neural Ensembles
Olfactory Coding and Decoding by Ensembles of Neurons
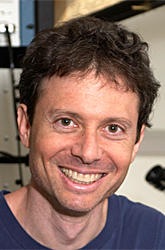
- Mark Stopfer, PhD, Head, Section on Sensory Coding and Neural Ensembles
- Zane Aldworth, PhD, Postdoctoral Fellow
- Nitin Gupta, PhD, Postdoctoral Fellow
- Takaaki Miyazaki, PhD, Postdoctoral Fellow
- Kazumichi Shimizu, PhD, Postdoctoral Fellow
- Samuel Reiter, MS, Graduate Student
All animals need to know what is going on in the world around them; thus, brain mechanisms have evolved to gather and organize sensory information in order to build transient and sometimes enduring internal representations of the environment. Using relatively simple animals and focusing primarily on olfaction, we combine electrophysiological, anatomical, behavioral, and other techniques to examine the ways in which intact neural circuits, driven by sensory stimuli, process information. In the past year, we investigated mechanisms, including transient oscillatory synchronization and slow temporal firing patterns of ensembles of neurons, that underlie information coding and decoding and how spontaneous activity arises in a sensory system, how it is regulated, and how innate sensory preferences are determined. Our work reveals basic mechanisms by which sensory information is transformed, stabilized, and compared as it makes its way through the nervous system.
Temporally diverse firing patterns in olfactory receptor neurons underlie neural codes for odors.
Odorants are represented as spatio-temporal patterns of spiking in the antennal lobes of insects and the olfactory bulbs of vertebrates. These odor-evoked ensemble responses, which contain information about odor identity, intensity, and timing, are reliable over repeated trials and are sometimes remarkably elaborate, consisting of sequences of excitation and inhibition that together can outlast the eliciting odor stimulus. The patterns change most rapidly during the odor's onset and offset. During the middle portions of lengthy odor presentations, the firings of populations of principal neurons tend to settle into stable patterns that have been described, in the language of dynamical systems analysis, as a "fixed point." Such activity patterns provide all the information that the brain receives about odors in the environment. The question is how the neural codes for odors are generated.
We hypothesized that the codes depend on a diversity of output from olfactory receptor neurons (ORN). To examine the characteristics of the input from the antenna to the antennal lobe, we first produced electro-antennograms (EAG) from isolated locust antennae; the EAG provides an assay of total ORN output. EAG responses evoked by different odorants all showed relatively similar time courses. Earlier models of antennal lobe function were based on input similar to these EAG patterns. However, given that the EAG sums population activity, it obscures any odor-specific temporal structure contributed by individual receptor neurons. To characterize the responses of individual ORNs that underlie the EAG response (and that provide olfactory input to the antennal lobe), we made the first systematic recordings from ORNs in the locust. We found that the responses of individual ORNs showed a surprising diversity of temporal structure, including periods of inhibition and sequences of excitation and inhibition. Viewed as a population, the response characteristics of the ORNs were in many ways remarkably similar to those observed downstream in the responses of the well-described projection neurons. Thus, many of the characteristics of odor codes thought to arise in the antennal lobe actually arise earlier in the periphery and include odor-elicited spatio-temporal patterning of principal neuron activity, decoupling of odor identity from intensity, and formation of fixed points for long odor pulses. The question arises as to the roles these complex peripheral responses might play in establishing neural codes for odors in the antennal lobe.
To examine systematically the significance of the diverse odor responses in the ORNs, we constructed a two-part computational model. The first part simulated a population of ORN odor responses while the second part realistically modeled the responses of the antennal lobe circuitry to the diversity of input. We found that our combined model generated responses that accurately matched the complexity, duration, and fixed-point characteristics of spatiotemporal odor codes recorded from the antennal lobe.
Further, given the surprising diversity and complexity of input provided by the receptor neurons, we considered what additional information-processing roles the antennal lobe might play. With our combined computational model, we found that the network dynamics of the antennal lobe imposes oscillatory synchronization, transforms the spatiotemporal input from ORNs into a higher-dimensional representation, and evenly redistributes odor codes to make better use of coding capacity. In several instances, our results demonstrated that apparently complex forms of information processing described in other systems may be explained by simple interactions between receptor neurons and their followers.
In view of the strong structural and functional parallels between insect and vertebrate olfactory systems, it seems likely that similar mechanisms operate across species. We therefore look forward to determining how ORNs generate diverse temporal responses to odors.
Spontaneous odor receptor neuron activity determines follower cell response properties.
Noisy or spontaneous activity poses a challenge to neural systems. Activity in the absence of obvious stimuli occurs throughout the central and peripheral nervous systems. Such spontaneous activity has been shown to play several useful roles; in some situations, however, it may not obviously benefit the organism but rather imposes limits on perception and behavior. We used the locust olfactory system to investigate fundamental properties of spontaneous activity at points along the sensory pathway. In insects, ORNs are distributed along the antenna. Our recordings from these neurons and their immediate and more distant followers revealed high levels of spontaneous activity in the ORNs themselves, local neurons, and projection neurons but little spontaneous activity in Kenyon cells of the mushroom body. Indeed, in a variety of species, spontaneous activity has been observed in ORNs and their immediate follower neurons (e.g., mitral cells in vertebrates); however, neurons one synapse farther (e.g., cortical neurons in vertebrates), such as Kenyon cells in insects, are typically almost silent at rest.
Where and how does this spontaneous activity originate? What rules govern its propagation from one group of neurons to the next, and how and why is such activity sharply limited two steps removed from the ORNs? What effects does spontaneous activity exert on olfactory coding? The locust olfactory system, where sensilla-containing ORNs are located both externally and accessibly, provided us with the opportunity to answer these questions directly.
We found that we could reversibly silence the ORNs by cooling them and that such silencing nearly abolished spontaneous and odor-elicited spiking in the projection neurons as well. Our investigation resolved a long-standing issue: spontaneous activity does not arise within the circuitry of the antennal lobe but rather is inherited entirely from the output of the ORNs. With a series of whole-cell patch-clamp recordings, we found that silencing the ORNs also significantly decreased the resting membrane potentials of projection neurons, local neurons, and Kenyon cells, indicating that spontaneous activity originating in the odor receptors exerts a constant influence on the response thresholds of follower cells.
To investigate the causes of spontaneous activity in ORNs, we controlled the delivery of odorant and the purity of air surrounding the antenna. In insects, as in vertebrates, absorbent fluid lymph surrounds ORNs. We found that isolating the antenna from its environment by coating it with an oily substance such as Vaseline® had little impact on spontaneous ORN output. However, manipulations designed to accelerate the removal of odorants from the sensillar lymph (surrounding the antenna with ultra-purified bottled air or directing high-speed streams of room air toward the antenna) significantly reduced the amount of output from the ORNs. Taken together, our results show that odorants or other ligands lingering in the sensillar lymph trigger spontaneous activity in the olfactory system. Environmental odorants continually presented to the antenna appear to play only a small role. Events within the ORNs' transduction machinery may make additional contributions to spontaneous activity.
To understand why spontaneous activity originating in ORNs is passed to the secondary projection neurons but is then sharply attenuated before reaching tertiary Kenyon cells, we developed a simple receiving operator characteristic (ROC) model to simulate the success of Kenyon cells in discriminating signal from noise, when presented with varying degrees of input convergence and ORN signal strength and various threshold set points. We found that, given the ongoing barrage of activity from ORNs, odor detection is optimal when projection neurons have a low response threshold; Kenyon cells, however, have a high response threshold. Our exploration of noise sources in the locust olfactory system provides a specific example of how a sensory system, bombarded with noise at the first stage of processing, balances the competing challenges of maintaining sensitivity to a wide range of stimuli and setting thresholds to eliminate noise and render neural codes more sparse. We expect that such strategies apply to other sensory systems that employ several stages of processing and circuitry convergence to achieve optimal detection.
Sensory neuron responses determine innate olfactory behaviors in the locust.
How do the sensory capacities of animals develop? Through their innate sensory preferences, animals often demonstrate the existence of inborn information. We are investigating how such information is encoded and how it differs from information acquired through direct experience. For our studies we use hatchling locusts, which offer several experimental advantages.
We found that newly hatched locusts, literally just crawling out of their egg hatching cups, immediately move toward fresh grass. In a series of behavioral studies using thousands of locusts, tested individually or in groups, we established that the hatchlings choose real grass over visually similar but odorless plastic grass; paper rubbed with fresh grass over clean paper of the same color; and paper dabbed with colorless monomolecular odorants that are components of grass odor over paper with other colorless odorants (even when the odorants were diluted to identical vapor pressures). The results indicate that naive locusts, which have never eaten, touched, or otherwise encountered their natural food source, have a built-in preference for its odor.
Such preference could be attributed to a peripheral mechanism; for example, hatchling locusts could have a surplus of odor receptors for grass odors. We tested this hypothesis by making electroantennograms from hatchlings and found that the antennae generate especially strong signals when stimulated by hexanol and octanol, which are volatile chemicals released by many green plants. Other odorants generated weaker responses. The same result obtained when we diluted the various odors to equal vapor pressure, indicating either that the hatchling and adult antennae contain a surfeit of hexanol and octanol receptors or that each hexanol and octanol receptor provides a particularly strong output. In addition, we found that, in hatchling antennae, sensory adaptation occurs for grass odors with the same timing and extent as for non-plant odors. Our results strongly suggest that the locust's innate sensory preference for grass odors is encoded peripherally, in the antenna. We also found that the response profiles of adult locust antennae are virtually identical to those of the new hatchlings, indicating that no experience or "tuning" is required to prepare locusts to detect grass odors preferentially.
Frequency shifts reveal basic mechanisms for odor-evoked neural oscillations.
Mounting evidence suggests that in many animals, from insects to mammals, olfactory information is represented by the temporally structured, synchronized firing of a spatially distributed population of neurons in the olfactory systems. We found that a wide range of odorants and stimulus pulse durations all evoked clear oscillatory activity in the moth Manduca sexta. Interestingly, relatively brief, plume-like pulses (shorter than 750 ms) produced only fast oscillations (30–40 Hz), whereas long pulses (longer than 1 s), such as those that effectively induce associative learning, produced an oscillatory burst that was initially fast and then slow (10–20 Hz). These results provide the first clear evidence that moths, like locusts, produce oscillatory responses to odors. The findings also provide the first indication that brief and lengthy presentations of the same odorant elicit somewhat different types of neural responses and that the oscillatory mechanism can operate stably in fast and slow modes.
What determines the oscillation frequency of a circuit, and how can a single neural circuit operate stably under two frequency regimes? We found that, in the moth, a lengthy odor pulse elicits an EAG deflection that, over the response, decreases in amplitude because of adaptation in ORNs. We also found that oscillation frequency roughly tracked EAG amplitude, suggesting that frequency may depend on the intensity of input to the circuitry of the antennal lobe.
However, we also discovered that, over a wide range of odor concentrations, the initial odor-elicited oscillation frequency was invariant, whereas EAG amplitudes varied greatly with concentration. Thus, some evidence suggested that oscillation frequency was dependent on input intensity, but other evidence suggested the opposite. To investigate this paradox, we developed a computational model of the moth antennal lobe that mimics the sharp transition between discrete fast and slow oscillatory states when input intensity gradually decreases. The recruitment of additional but less well-tuned ORNs to simulate responses to higher concentrations did not affect oscillation frequency. Our recordings from ORNs showed that long odor pulses caused most individual ORNs to adapt their firing rates rapidly; the change in firing rate closely matched the time course of the shift in oscillation frequency. Our results suggest that oscillation frequency can shift between two stable states, depending on the varying output intensity of adapting receptors rather than on odor concentration. Our model indicates that such a shift in oscillation frequency is possible if the ORNs that are highly tuned for a given odor fire at near-saturating rates, even when presented with low odor concentrations; higher concentrations recruit additional but less tuned ORNs. The model also revealed that oscillation frequency is regulated flexibly by the intensity of input rather than rigidly by the duration of the inhibitory post-synaptic potential from inhibitory neurons, as is often assumed.
Several mechanisms extract features from natural odor stimuli.
As information moves through the brain, it undergoes dramatic transformation in myriad ways. Our previous work suggested that one of the general mechanisms responsible for such transformation is neural plasticity. To investigate mechanisms underlying the transformations, we delivered rapid, repeated pulses of odors with timing designed to mimic features of natural plumes and monitored, in intact animals, neural activity in several locations: olfactory receptor neurons, ensembles of projection and local first-order interneurons of the antennal lobe (analogous to the olfactory bulb), and the second-order Kenyon cells of the mushroom body (analogous to the pyriform cortex). At each location, we sought to understand responses in terms of the interactions of plasticity occurring at earlier sites. We also sought to understand the transformations' potential value to the animal.
We found that interneuronal responses to natural odor stimuli are shaped by at least two plastic mechanisms: rapid adaptation in the receptors and relatively enduring facilitation of inhibition within the receptors' downstream targets. Peripheral adaptation renders the olfactory system relatively insensitive to stimuli that repeat very rapidly. Central facilitation of inhibition increases the reliability and sparseness of stimuli that are encountered repeatedly but are separated by long intervals. Further, these mechanisms constrain the projection neuron ensemble in order to provide relatively stable output to its downstream followers, thereby permitting the encoding of information about odor identity and concentration with firing patterns that are not confounded by the timing patterns of the stimulus.
How do the follower neurons decode this time-varying ensemble activity? Intracellular and extracellular recordings from Kenyon cells showed that the cells' firing rates change dramatically throughout trains of odor pulses in a timing-dependent manner; for brief inter-pulse intervals, the great majority of action potentials fire at the beginning of the train and again following the train's conclusion. We found that the Kenyon cells' firing threshold may be met when projection neurons fire at rates that are relatively low but with spikes that are highly synchronized across the population by the oscillatory mechanism of the antennal lobe (as occurs during the onset of the pulse train). On the other hand, the threshold may be met when the instantaneous firing rate of the projection neuron ensemble is high in the absence of pronounced synchronization (as occurs following the offset of the train).
Together, our work suggests that the non-associative plasticity elicited by odor plumes leads to responses in the projection neuron ensemble that combine an instantaneous report of sensory input with a record of recent input, allowing the extraction of high-level features.
Additional Funding
- Japan Society for the Promotion of Science fellowship to Takaaki Miyazaki
Publications
- Ito I, Bazhenov M, Ong R, Raman B, Stopfer M. Frequency transitions in odor-evoked neural oscillations. Neuron 2009;64:692-706.
- Assisi C, Stopfer M, Bazhenov M. Using the structure of inhibitory networks to unravel mechanisms of spatiotemporal patterning. Neuron 2011;69:373-386.
- Raman B, Joseph J, Tang J, Stopfer M. Temporally diverse firing patterns in olfactory receptor neurons underlie spatiotemporal neural codes for odors. J Neurosci 2010;30:1994-2006.
- Tanaka NK, Dye L, Stopfer M. Dual-labeling method for electron microscopy to characterize synaptic connectivity using genetically encoded fluorescent reporters in Drosophila. J Neurosci Meth 2010;194:312-315.
- Raman B, Stopfer M. Analysis of trial-by-trial variability in stimulus-evoked neural activity. Conference Proceedings of IEEE Engineering in Medicine and Biology Society (EMBC) 2010;1:4320-4322.
Collaborators
- Maxim Bazhenov, PhD, Howard Hughes Medical Institute, The Salk Institute for Biological Studies, La Jolla, CA
- Luca A. Finelli, PhD, Howard Hughes Medical Institute, The Salk Institute for Biological Studies, La Jolla, CA
- Kei Ito, PhD, University of Tokyo, Tokyo, Japan
- Terrence J. Sejnowski, PhD, Howard Hughes Medical Institute, The Salk Institute for Biological Studies, La Jolla, CA
Contact
For more information, email stopferm@mail.nih.gov or visit neuroscience.nih.gov/Faculty/Profile/mark-stopfer.aspx.