You are here: Home > Section on Molecular Transport
Biophysics of Large Membrane Channels
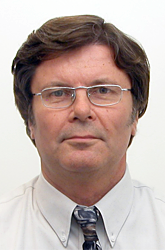
- Sergey M. Bezrukov, PhD, Head, Section on Molecular Transport
- Tatiana K. Rostovtseva, PhD, Staff Scientist
- Oscar Teijido Hermida, PhD, Visiting Fellow
- Shay M. Rappaport, PhD, Visiting Fellow
- Kely L. Sheldon, MS, Predoctoral Intramural Research Training Award Fellow
To address fundamental questions of membrane transport, we focus on the mechanisms that govern channel-facilitated permeation of metabolites and other large solutes across cell and organelle membranes. In particular, we concentrate on mitochondrial and bacterial membrane proteins that produce “large” mostly beta-barrel channels. As our main tools, we combine approaches of theoretical physics with experiments that allow us to investigate the channel-forming proteins in their functional states by using channel reconstitution into planar lipid bilayers. The channels are recognized not only as the gateways of metabolite exchange between different cellular compartments and cells, but also as multifunctional membrane receptors and components of many toxins, which are beginning to emerge as novel drug targets.
The channel-forming proteins and peptides we work with include VDAC (voltage-dependent anion channel from the outer membrane of mitochondria), alpha-Hemolysin (toxin from Staphylococcus aureus), translocation pores of Bacillus anthracis (PA63), Clostridium botulinum (C2IIa), and Clostridium perfringens (Ib) binary toxins, Epsilon toxin (from Clostridium perfringens), OmpF (general bacterial porin from Escherichia coli), LamB (sugar-specific bacterial porin from Escherichia coli), OprF (porin from Pseudomonas aeruginosa), Syringomycin E (lipopeptide toxin from Pseudomonas syringae), and the bacterial peptide TisB involved in persister cell biofilm formation. We also use Gramicidin A (linear pentadecapeptide from Bacillus brevis) as a molecular sensor of membrane mechanical properties.
To study channels under precisely controlled conditions, we first isolate the channel-forming proteins from their host organisms, purify them, and then reconstitute them into planar lipid bilayer membranes. Our main goal is to elucidate the physical principles and molecular mechanisms that control metabolite flux under normal and pathological conditions. Specifically, we study channel interactions with the lipid membrane as modified by volatile anesthetics, with cytosolic proteins, and with newly synthesized drugs, such as blockers of the translocation pores of bacterial toxins. By learning the physics, chemistry, and physiology of channel functioning, we strive to determine how to design new agents and strategies that effectively correct the deviant interactions associated with disease.
Interactions of high-affinity cationic blockers with the translocation pores of B. anthracis, C. botulinum, and C. perfringens binary toxins
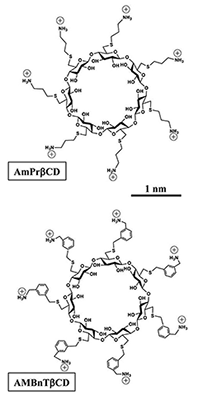
Click image to enlarge.
Figure 1.
Two sevenfold symmetrical synthetic molecules, AmPrßCD (top panel) and AMBnTßCD (bottom panel), were used as blockers of the PA63, C2IIa, and Ib pores (B components of anthrax, C2, and iota toxins, respectively).
Recently, we introduced cationic beta-cyclodextrin derivatives as highly effective and potentially universal blockers of three binary bacterial toxins: anthrax toxin of Bacillus anthracis, C2 toxin of Clostridium botulinum, and iota toxin of Clostridium perfringens. The binary toxins consist of two separate components: the enzymatic A component, which acts on certain intracellular targets, and the binding/translocation B component, which forms oligomeric channels in the target cell membrane. This year, in collaboration with scientists from Institut Pasteur, the University of Ulm Medical Center, NIAID, and Innovative Biologics, we studied the voltage and salt dependence of the rate constants of binding and dissociation reactions of two structurally distinct beta-cyclodextrins (AmPrβCD and AMBnTβCD, Figure 1) with the PA63, C2IIa, and Ib channels (B components of anthrax, C2, and iota toxins, respectively). With all three channels, AMBnTβCD, the blocker carrying extra hydrophobic aromatic groups on the thio-alkyl linkers of positively charged amino groups, demonstrated significantly stronger binding than did AmPrβCD. The effect was seen as an increased residence time of the blocker in the channels, whereas the time between blockages characterizing the binding reaction on-rate remained virtually unchanged. Surprisingly, the voltage sensitivity, expressed as a slope of the logarithm of the blocker residence time as a function of voltage, was more or less the same for all six cases studied, suggesting structural similarities among the three channels. Also, the more effective AMBnTβCD blocker shows weaker salt dependence of the binding and dissociation rate constants than does AmPrβCD. By estimating the relative contributions of the applied transmembrane field and of long-range Coulomb and salt concentration–independent short-range forces, we found that the latter represent the leading interaction, which accounts for the high efficiency of blockage. In a search of the putative groups in the channel lumen responsible for the short-range forces, we performed measurements with the F427A mutant of PA63, which lacks the functionally important phenylalanine clamp. We found that the on-rates of the blockage were virtually conserved but that the residence times and, correspondingly, the binding constants dropped by more than an order of magnitude, which also reduced the difference between the efficiencies of the two blockers. Thus, our quantitative analysis allowed us to discriminate between the physical forces defining the strength of interactions and, therefore, to help in a directed search for efficient antidotes.
VDAC regulation by tubulin: role of the membrane
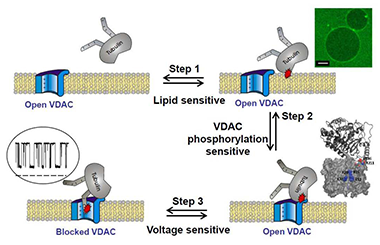
Click image to enlarge.
Figure 2.
VDAC blockage by tubulin involves multiple steps. One of the critical steps is tubulin interaction with the membrane. The cartoon includes images of tubulin binding to DOPE–containing GUVs (scale bar is 10 mm), MD simulations of VDAC putative cytosolic loops of tubulin binding, and a current trace of tubulin-induced VDAC blockage.
Elucidating molecular mechanisms by which lipids regulate protein function within biological membranes is critical for understanding many cellular processes. The ability of lipids to regulate proteins arises from both specific chemical features of lipid molecules and mechanical and structural properties of the lipid bilayer. Structural, compositional, and elastic parameters of lipid membranes are known to exert a strong influence on the function of membrane proteins, such as ion channels, as well as on the interaction of water-soluble proteins with membranes. Recently, we have found that dimeric tubulin, a subunit of microtubules, regulates mitochondrial respiration by blocking the VDAC of the mitochondrial outer membrane. This past year, we showed that the mechanism of VDAC blockage by tubulin involves, as a critical step, tubulin interaction with the membrane. The on-rate of the blockage varies up to 100-fold, depending on the lipid composition used for bilayer formation in reconstitution experiments, and rises with increasing content of dioleoylphosphatidylethanolamine (DOPE) in dioleoylphosphatidylcholine (DOPC) bilayers. At physiologically low salt concentrations, the on-rate is reduced by the charged lipid. The off-rate of VDAC blockage by tubulin does not depend on the lipid composition. Using confocal fluorescence microscopy, we compared tubulin binding to the membranes of giant unilamellar vesicles (GUVs) made from DOPC and DOPC/DOPE mixtures. We found that detectable binding of the fluorescently labeled dimeric tubulin to GUV membranes requires the presence of DOPE. We propose that, prior to the characteristic blockage of VDAC, tubulin first binds to the membrane in a lipid-dependent manner (Figure 2). We thus revealed a new potent regulatory role of the mitochondrial lipids in controlling mitochondrial outer membrane permeability, and hence mitochondrial respiration, through tuning VDAC sensitivity to blockage by tubulin. More generally, our findings provide an example of lipid-controlled protein-protein interaction where the choice of lipid species is able to change the equilibrium binding constant by orders of magnitude.
Physical theory of facilitated transport
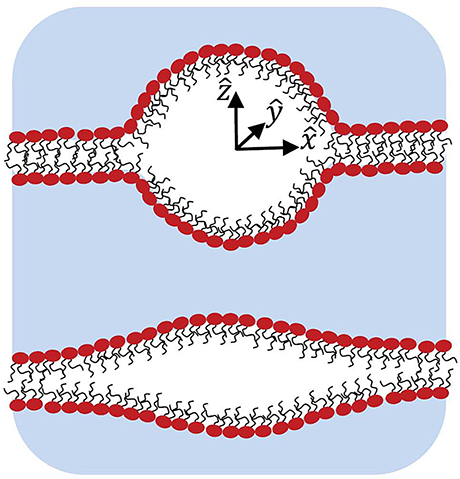
Click image to enlarge.
Figure 3.
Schematic illustration of cross sections of two intra-membrane cavities shows a mostly spherical shape with sharp edges but relatively small surface area (upper image) and the stretched one with smoother edges but relatively large surface area (lower image). One of the goals of the study was to find an optimal cavity shape at a given volume and then to analyze its energetics.
This year, we focused on two topics: (i) the effects of channel/receptor clustering and (ii) intra-membrane cavitation as a mechanism of membrane permeabilization. Various membrane functional units such as receptors, transporters, and channels, whose action necessarily involves capturing diffusing molecules, are often organized into multimeric complexes forming clusters on the cell and organelle membranes. These functional units themselves are usually oligomers of several integral proteins, which have their own symmetry. Depending on the symmetry, they form clusters on different packing lattices. Moreover, local membrane inhomogeneities, e.g., the so-called membrane domains, rafts, stalks, etc., lead to different patterns even within the structures on the same packing lattice. It is clear that the units located at the cluster periphery partially screen the units in the central part of the cluster from diffusing molecules. We were able to formulate a general approach that allows one to quantitatively describe the screening effects. The approach is used to derive simple expressions giving the trapping rates of diffusing molecules by clusters of absorbers on lattices of various packing symmetries. Our analysis shows how the trapping rate changes from the sum of the rates of individual absorbers forming the cluster to the declining effective collective trapping rate as the number of absorbers in the cluster increases and/or the inter-absorber distance decreases. Numerical tests demonstrate good agreement between the rates predicted by the theory and those obtained from Brownian dynamics simulations for clusters of different shapes, lattice symmetries, and sizes.
The study of intra-membrane cavitation (IMC) was motivated by recent publications suggesting that this type of cavitation, rather than homogeneous cavitation, is crucial in ultrasound-induced membrane permeabilization and traumatic brain injury. We focused on the thermodynamics of IMC, namely, on the minimum work required to form an intra-membrane cavity. The minimum work can be separated into two parts, one that depends on the volume and number of gas molecules in the bubble and another that depends on the bubble geometry. Using a simplified assumption about the cavity shape, the geometry-dependent term is derived and minimized at a fixed cavity volume. We found that the optimized cavity is almost spherical at large bubble volumes, while at small volumes the cavity has a lens-like shape (Figure 3). The optimized shape was used to analyze the minimum work of IMC, which turned out to be significantly smaller than that of the homogeneous cavitation, thus suggesting a leading role for IMC in the formation of membrane defects during ultrasound-induced membrane permeabilization and in traumatic brain injury.
Additional Funding
- NRC grant “Developing a bio-mimetic activating platform for the mitochondrial outer membrane” from NIH(NIBIB)/NIST Joint Sponsorship Program (2013-2015).
Publications
- Bezrukov SM, Liu X, Karginov VA, Wein AN, Leppla SH, Popoff MR, Barth H, Nestorovich EM. Interactions of high-affinity cationic blockers with the translocation pores of B. anthracis, C. botulinum, and C. perfringens binary toxins. Biophys J 2012;103:1208-1217.
- Rostovtseva TK, Gurnev PA, Chen M-Y, Bezrukov SM. Membrane lipid composition regulates tubulin interaction with mitochondrial voltage-dependent anion channel. J Biol Chem 2012;287:29589-29598.
- Berezhkovskii AM, Dagdug L, Vazquez M-V, Lizunov VA, Zimmerberg J, Bezrukov SM. Trapping of diffusing particles by clusters of absorbing disks on a reflecting wall with disk centers on sites of a square lattice. J Chem Phys 2013;138:064105.
- Rappaport SM, Berezhkovskii AM, Zimmerberg J, Bezrukov SM. Thermodynamics of inter-leaflet cavitation in lipid bilayer membranes. Phys Rev E 2013;87:022715.
- Gurnev PA, Yang S-T, Melikov KC, Chernomordik LV, Bezrukov SM. Cationic cell-penetrating peptide binds to planar lipid bilayers containing negatively charged lipids but does not induce conductive pores. Biophys J 2013;104:1933-1939.
Collaborators
- Vicente M. Aguilella, PhD, Universidad Jaume I, Castellón, Spain
- Holger Barth, PhD, Institute of Pharmacology and Toxicology, Universität Ulm, Ulm, Germany
- Alexander M. Berezhkovskii, PhD, Division of Computational Bioscience, CIT, NIH, Bethesda, MD
- Susan K. Buchanan, PhD, Laboratory of Molecular Biology, NIDDK, Bethesda, MD
- Leonid V. Chernomordik, PhD, Program in Physical Biology, NICHD, Bethesda, MD
- Leonardo Dagdug, PhD, Universidad Autónoma Metropolitana-Iztapalapa, Mexico City, Mexico
- Vladimir A. Karginov, PhD, Innovative Biologics, Herndon, VA
- John J. Lemasters, PhD, Medical University of South Carolina, Charleston, SC
- Ekaterina M. Nestorovich, PhD, The Catholic University of America, Washington, DC
- Sergei Y. Noskov, PhD, University of Calgary, Canada
- Adrian Parsegian, PhD, University of Massachusetts Amherst, Amherst, MA
- Michel R. Popoff, PhD, Department of Host-Pathogen Interactions, Institut Pasteur, Paris, France
- Dan Sackett, PhD, Program in Physical Biology, NICHD, Bethesda, MD
- Gerhard Wagner, PhD, Harvard Medical School, Boston, MA
- Michael Weinrich, MD, National Center for Medical Rehabilitation Research, NICHD, Bethesda, MD
- David L. Worcester, PhD, National Institute of Standards and Technology. Gaithersburg, MD
- Josh Zimmerberg, MD, PhD, Program in Physical Biology, NICHD, Bethesda, MD
Contact
For more information, email bezrukov@helix.nih.gov or visit smt.nichd.nih.gov.