You are here: Home > Section on Cell Biophysics
Cell Biophysics
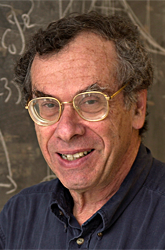
- Ralph Nossal, PhD, Head, Section on Cell Biophysics
- Dan Sackett, PhD, Staff Scientist
- Anand Banerjee, PhD, Visiting Fellow
- Felipe Montecinos, PhD, Visiting Fellow
- Raimon Sunyer, PhD, Visiting Fellow
- Hacène Boukari, PhD, Guest Researcher
- Norman Gershfeld, PhD, Guest Researcher
- Matthew Mirigian, BA, Guest Researcher
- Silviya Zustiak, PhD, Guest Researcher
Our investigations center on how cell behavior is linked to underlying physical mechanisms, for which we develop and apply methodologies based on mathematical and physical principles. Our research also involves biochemical and cell-biological techniques. Projects currently include: (i) elaborating a physical model to explain the stochastic nature of coated vesicle biogenesis during receptor-mediated endocytosis, focusing on understanding how the size dependence of nanoparticle uptake relies on mesoscopic cell mechanics; (ii) using atomic force spectroscopy to examine the modes by which clathrin lattices can be dissociated; (iii) exploring how substrate mechanical properties affect the response of eukaryotic cells to changes in their environment; and (iv) understanding how certain small molecules interact with microtubules and thereby act as antimitotic agents, and how microtubule arrays function in mitosis to produce accurate segregation of chromosomes. We also develop new experimental modalities to characterize these and related phenomena. We have a particular interest in the ways cellular activities are coordinated in space and time.
Complex systems biophysics
We have been employing physical and mathematical methods to understand the biophysics of complex cellular processes. For example, we study the biogenesis of coated vesicles involved in clathrin-mediated endocytosis (CME) and other intracellular transport processes. CME is the principal pathway for the regulation of receptors and the internalization of certain nutrients and signaling molecules at the plasma membrane of eukaryotic cells. The early stage of receptor-mediated endocytosis involves the formation of transient structures known as clathrin-coated pits (CCPs) which, depending on the detailed energetics of protein binding and associated membrane transformations, either mature into clathrin-coated vesicles (CCVs) or regress and vanish from the cell surface. The former are referred to as “productive” CCPs and the latter as "abortive" CCPs. We previously developed a simple physical model for CCP dynamics and carried out Monte Carlo simulations to investigate the time development of CCP size, explain the origin of abortive pits, and reproduce features of their lifetime distribution. By fitting our results to experimental data, we estimated values of the free energy changes involved in formation of the protein coat that surrounds a CCV, and we showed how the binding of cargo might modify the coat parameters and thereby facilitate CCV formation (1). We also derived analytical expressions for various characteristic quantities, including the lifetime distribution and the distribution of maximum sizes of abortive pits, the probability that, once started, a CCP is productive, and the mean time for the growth of a CCP to the point where it is released from the plasma membrane. Using these expressions, we then calculated the mean time between the binding of a nanoparticle (NP) to a cell surface and its internalization as a result of CME. The approach enabled us to show that NP internalization occurs most efficiently when NP size is commensurate with the natural curvature of the clathrin-containing protein coat of the vesicle.
We also have been developing predictive models to illuminate other aspects of receptor-mediated endocytosis (RME), of which CME is the best characterized. For example, RNAi depletion experiments indicate that a well defined threshold value of intracellular clathrin must be exceeded before CCVs are produced and that empty clathrin cages are rarely seen in the cytoplasm. To understand these observations, we used a thermodynamic model to investigate the conditions under which clathrin triskelions form polyhedral baskets. The analysis, which is similar to classical methods used to study micelle formation, relates clathrin-basket energetics to system parameters that are linked to triskelial rigidity, the natural curvature of an isolated triskelion, and interactions between triskelial legs in the assembled polyhedra. The theory predicts that a minimal ("critical") concentration must be surpassed in order for basket polymerization to occur and indicates how this quantity and the amount of polymerized material depend on the chosen parameters (2). In particular, we obtained a general mathematical expression that relates the critical concentration to the free energy change that occurs when baskets of maximal prevalence assemble. Using explicit expressions for this quantity, we confirmed that the critical concentration is lowered by factors that increase basket stability. Various cell processes compete for clathrin, including interactions with microtubule-binding proteins during mitosis and processes occurring at endosomes and the Golgi. Thus, critical concentration biophysics may be an important factor in the global control of cell activities involving clathrin.
Our research also involves adapting physical methods such as dynamic light scattering, small angle neutron scattering, and atomic force spectroscopy to infer the physical properties of the biochemical entities involved in RME. In our recent work, we used atomic force microscopy (AFM) and single-molecule force spectroscopy (SMFS) to characterize intermolecular interactions and domains of clathrin triskelions and their assemblies (3). To assess triskelion structure and triskelion-triskelion interactions, we subjected purified individual triskelions, bovine-brain CCVs, and reconstituted clathrin-AP180 coats (AP180 is one of many assembly proteins and adaptors for clathrin) to AFM-SMFS pulling experiments and applied newly derived analytics to extract force-extension relations from very large data sets. For individual triskelions, SMFS revealed a series of unfolding events associated with individual heavy chain alpha-helix hairpins containing ca. 30 amino acid residues. Cooperative unraveling of several hairpin domains up to the size of the known repeating motif of ca. 145 amino acid residues also was seen. We found that the clathrin lattices of AP180–mediated coats are energetically easier to unravel than those of native CCVs. Studies of such clathrin assemblies expose weaker, but coordinated, clathrin-clathrin interactions, which are indicative of the inter-leg associations essential for clathrin-mediated endocytosis. The investigation is a continuation of our earlier work to establish various mechanical characteristics of clathrin structures, given that such properties are important elements in physical models such as those mentioned above.
Tubulin polymers and cytoskeletal organization
We continued our study of the properties of microtubules (MT), and of drugs that alter these properties, and of the biology of MT arrays, such as the mitotic spindle. Our recent work identified the binding site for a new microtubule-stabilizing drug. In the course of this work, we needed to revisit the biophysical methods used for monitoring drug effects on the polymerization of MT in vitro. In addition, we addressed some mitosis-specific proteins that affect the fidelity of mitosis and which may be important in generating chromosome defects seen in cancer.
Polymerization of MT from purified tubulin, the major structural protein in MT, is an accepted model for quantitatively evaluating the effects of established MT–targeting drugs, as well as for screening collections of compounds for new MT–targeting agents. The most common method is to monitor the increase in light scattering produced by inducing a solution of tubulin to polymerize into MT. This is usually measured with a simple spectrophotometer, using longwave UV light (typically 350 nm). While an increase provides an unambiguous signal of the formation of larger structures in the solution, the mere fact of increased light scattering does not mean that the larger structures are MT; random aggregation would also produce an increase in signal. We demonstrated how a slightly more careful approach to this method than is commonly used will yield much more information, including a rough check that the polymers produced are MT. We also combined this with fluorescence methods for monitoring polymerization that are somewhat more resistant to the effects of aggregation (4).
MT–targeting drugs induce arrest of mitosis in rapidly dividing cells but also alter MT function during the 95% or more of the time that a cell is not in mitosis. The latter functions are likely the reason that such drugs are effective chemotherapy agents against many human cancers. Even if mitosis is not the main target of these drugs in tumors, as seems to be the case, it is nonetheless clear that cancer cells accumulate many chromosomal defects that originate in faulty mitosis. One such common defect is aneuploidy, in which cells have abnormal numbers of chromosomes, likely a result of problems in the functional fidelity of the mitotic spindle. The mitotic spindle is a complicated dynamic structure, composed of a pair of MT arrays, which is responsible for aligning the chromosomes and then accurately separating them so that each daughter cell receives one copy of each chromosome. Cells have several mechanisms to avoid dysfunction of the mitotic spindle, some of which involve proteins that are only expressed and active during mitosis. The expression of one of these proteins, CKAP2, is altered in some cancers. We studied the protein to understand how it helps stabilize mitotic spindle function and how alterations in its expression in cells can lead to aneuploidy in the daughter cells (5). We discovered that CKAP2 regulates the functioning of the two poles of the MT arrays in the spindle. Abnormal amounts of CKAP2 reduce the ability of cells to form two and only two spindle poles, a condition necessary for normal spindle structure. When there are several poles, it is impossible to divide chromosomes evenly between two daughter cells. Such a failure, or deficit, of CKAP2 function leads to attachment failure in some fraction of the chromosomes and to accumulation of aneuploidy in the cell population.
Biophysical methods and models
We continue to develop novel physics-based methodologies for investigating complex biological structures and materials. One of our activities involved studying the effects of multiple scattering on the interpretation of fluorescence correlation spectroscopy (FCS) measurements performed on optically dense samples. A unique power of FCS is that it can detect the motions of fluorescent entities while signals from non-fluorescent surroundings can be ignored. For this reason, FCS is being used increasingly to study particles located in a complex milieu; examples are molecules moving on cell surfaces, antibiotics and viruses diffusing in biofilms, and morphogens moving within embryos. Using well defined scattering models, we investigated the reliability of parameters determined by FCS when it is used to probe molecules in such environments. For example, we measured FCS auto-correlation functions of Atto 488 dye molecules diffusing in solutions of polystyrene beads, which act as scatterers. As much as a two-fold, scattering-linked increase in the illuminated volume was noted, but only a minimal increase in apparent diffusivity was evident. We employed Monte-Carlo simulations to analyze the illuminated beam profile, finding that beam broadening occurs primarily along the axial direction. However, the increased volume in the axial direction has only a negligible effect on the measured diffusion time because intensity fluctuations arising from diffusion in the radial direction are dominant in FCS measurements. Although a reduction of the incident intensity happens at the focal point, our results indicate that significant measurement artifacts do not occur, implying that single-photon FCS can be used to probe diffusivity in optically dense media when sufficient signal intensity is attainable. The results have informed work in which we employ FCS to investigate the diffusion of macromolecules and other targets within the fluid phase of polymer gels and in concentrated polymer solutions. A current application is a study of the diffusion of growth factors and chemokines within agarose gels of differing polymer concentrations, undertaken in conjunction with mathematical analysis of an "agarose spot" assay for the chemotactic response of cancer-derived tissue culture cells to such signaling molecules.
In addition, we recently carried out two projects related to mechanical aspects of cell response. Both were directed at establishing reliable methods for assessing the coupling between substrate properties and fundamental cell processes such as angiogenesis, neurogenesis, and cancer metastasis, which are thought to be modulated by extracellular matrix stiffness. In the first, we developed a method to fabricate bio-compatible hydrogels with well defined linear stiffness gradients. The method, involving photopolymerization of films by progressively uncovering an acrylamide/bis-acrylamide solution initially covered with an opaque mask, can be easily implemented with common lab equipment. It produces linear stiffness gradients of at least 40 kPa/mm, extending from less than 1 kPa to 80 kPa (in units of shear modulus). Hydrogels with less steep gradients and narrower stiffness ranges can also be easily produced. The hydrogels can be covalently functionalized with uniform coatings of proteins that promote cell adhesion. Cell spreading on these hydrogels correlates linearly with hydrogel stiffness, indicating that the technique can be used to study the motile, mechanical response of living cells. Potential applications are in studies of wound healing and development of embryonic tissues.
It is becoming increasingly clear that the cell microenvironment, including the surrounding matrix, profoundly affects cell fate. This is especially true for solid tumors in which matrix stiffness, for example, is believed to be an important factor in tumorogenesis. Given that matrix stiffness affects cell fate, it may also be important in drug resistance. Consequently, we undertook a second project in which we designed and built a multiwell, “high-throughput,” polyacrylamide (PA) gel–based stiffness assay. The gels were coated with collagen in order to facilitate cell attachment and proliferation, and the polyacrylamide substrates were fabricated with stiffness values ranging from 0.3 to 300 kPa. The high-throughput format facilitates obtaining dose-response curves through simultaneous testing of several parameters. Additionally, the assay is an improvement upon other techniques in terms of preparation time, robustness, and cost. We thereby examined the effect of stiffness on cultured cell responsiveness to cytotoxic drugs. In particular, we demonstrated the utility of the assay by testing multiple cancer cell lines and their susceptibility to paclitaxel, a microtubule-targeting agent.
Publications
- Banerjee A, Berezhkovskii A, Nossal R. Stochastic model of clathrin-coated pit assembly. Biophys J 2012;102:2725-2730.
- Muthukumar M, Nossal, R. Micelle-like model for the polymerization of clathrin baskets. J Chem Phys 2013;139:121928.
- Jin AJ, Lafer EM, Peng JG, Smith PD, Nossal R. Unraveling protein-protein interactions in clathrin assemblies via atomic force spectroscopy. Methods 2013;59:316-327.
- Mirigian M, Mukherjee K, Bane SL, Sackett DL. Measurement of in vitro microtubule polymerization by turbidity and fluorescence. Methods Cell Biol 2013;115:215-229.
- Case CM, Sackett DL, Wangsa D, Karpova T, McNally JG, Ried T, Camps J. CKAP2 ensues chromosomal stability by maintaining the integrity of microtubules. PLoS One 2013;8:e64575.
Collaborators
- Susan Bane, PhD, Binghamton University, Binghamton, NY
- Alexander Berezhkovskii, PhD, Division of Computational Bioscience, CIT, NIH, Bethesda, MD
- Hacène Boukari, PhD, Delaware State University, Dover, DE
- Tito Fojo, MD, PhD, Medical Oncology Branch, NCI, Bethesda, MD
- Amir Gandjbakhche, PhD, Program in Pediatric Imaging and Tissue Sciences, NICHD, Bethesda, MD
- Jeeseong Hwang, PhD, National Institute of Standards and Technology, Boulder, CO
- Albert J. Jin, PhD, Laboratory of Cellular Imaging and Macromolecular Biophysics, NIBIB, Bethesda, MD
- Eileen Lafer, PhD, University of Texas Health Science Center, San Antonio, TX
- Naomi Morrissette, PhD, University of California Irvine, Irvine, CA
- Muraggapan Muthukumar, PhD, University of Massachusetts, Amherst, MA
- John Park, MD, Surgical Neurology Branch, NINDS, Bethesda, MD
- Dave Schriemer, PhD, University of Calgary, Calgary, Canada
- David Sept, PhD, University of Michigan, Ann Arbor, MI
- Richard Taylor, PhD, Notre Dame University, Notre Dame, IN
- Karl Werbovetz, PhD, Ohio State University, Columbus, OH
- Al Yergey, PhD, Mass Spectrometry Core Facility, NICHD, Bethesda, MD
Contact
For more information, email nossalr@mail.nih.gov.