You are here: Home > Section on Protein Biosynthesis
Mechanism and Regulation of Eukaryotic Protein Synthesis
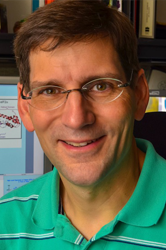
- Thomas E. Dever, PhD, Head, Section on Protein Biosynthesis
- Byung-Sik Shin, PhD, Staff Scientist
- Ivaylo P. Ivanov, PhD, Research Fellow
- Margarito Rojas, PhD, Visiting Fellow
- Chune Cao, Biological Laboratory Technician
- Erik Gutierrez, BS, Graduate Student
- Jason A. Murray, BS, Graduate Student
- Joo-Ran Kim, BS, Special Volunteer
We study the mechanism and regulation of protein synthesis, focusing on GTPases and protein kinases that control this fundamental cellular process. We use molecular-genetic and biochemical studies to dissect the structure-function properties of the translation initiation factors eIF2, a GTPase that binds methionyl-tRNA to the ribosome, and eIF5B, a second GTPase that catalyzes ribosomal subunit joining in the final step of translation initiation. We also investigate stress-responsive protein kinases that phosphorylate eIF2alpha, viral regulators of these kinases, and how cellular phosphatases are targeted to dephosphorylate eIF2alpha. Our recent studies elucidated how an eIF2gamma mutation that is associated with intellectual disability impairs eIF2 function and revealed how eIF2 binds to methionyl-tRNA and the ribosome. Our studies also demonstrated that the hypusine-containing protein eIF5A promotes translation elongation by stimulating the peptidyl transferase activity of the ribosome and facilitating the reactivity of poor substrates such as proline.
Molecular analysis of eIF2alpha phosphorylation, dephosphorylation, and viral regulation
The translation factor eIF2 is composed of three distinct subunits. Phosphorylation of the eIF2alpha subunit is a common mechanism for downregulating protein synthesis under stress conditions. Four distinct kinases phosphorylate eIF2alpha on Ser51 under different cellular stress conditions. GCN2 responds to amino acid limitation, HRI to heme deprivation, PERK to ER stress, and PKR to viral infection. Consistent with their common activity to phosphorylate eIF2alpha on Ser51, the kinases show strong sequence similarity in their kinase domains. Phosphorylation of eIF2alpha converts eIF2 from a substrate to an inhibitor of its guanine-nucleotide exchange factor eIF2B. The inhibition of eIF2B impairs general translation, slowing the growth of yeast cells and, paradoxically, enhancing the translation of the GCN4 mRNA required for yeast cells to grow under amino-acid starvation conditions.
We previously used structural, molecular, and biochemical studies to define how the eIF2alpha kinases recognize their substrate. In collaboration with Frank Sicheri, we obtained the X-ray structure of eIF2alpha bound to the catalytic domain of PKR. Back-to-back dimerization enables each PKR protomer to engage a molecule of eIF2alpha in the crystal structure. Using site-directed mutagenesis studies, we demonstrated that a common mode of back-to-back dimerization is required for activation of PKR, GCN2, and PERK, and we proposed an ordered mechanism of PKR activation by which catalytic domain dimerization triggers autophosphorylation, which in turn is required for specific eIF2alpha substrate recognition. Our mutagenesis studies revealed that the position of the Ser51 residue in free eIF2alpha is restricted and that docking of eIF2alpha onto PKR helix alphaG disrupts a hydrophobic network and induces a conformational change that enables Ser51 to move by about 20 Å to engage the phospho-acceptor binding site of the kinase. We propose that the protected state of Ser51 in free eIF2alpha prevents promiscuous phosphorylation and the attendant translational regulation by heterologous kinases, yet enables Ser51 phosphorylation upon binding of eIF2alpha to one of the canonical eIF2alpha kinase.
As part of the mammalian cell's innate immune response, the double-stranded RNA (dsRNA)–activated protein kinase PKR phosphorylates the translation initiation factor eIF2alpha to inhibit protein synthesis and thus block viral replication. To subvert this host cell defense mechanism, viruses produce inhibitors of PKR. Several members of the poxvirus family express two distinct types of PKR inhibitor: a pseudosubstrate inhibitor (such as the vacciniavirus K3L protein that resembles the N-terminal third of eIF2alpha) and a dsRNA-binding protein called E3L. High-level expression of human PKR inhibited the growth of yeast, and co-expression of the vacciniavirus K3L or E3L protein (or the related variolavirus [smallpox] C3L or E3L protein, respectively) restored yeast cell growth. We are currently characterizing mutations in PKR that confer resistance to E3L inhibition and are studying the functional domains of the E3L protein. The E3L protein contains an N-terminal Z-DNA–binding (Zalpha) domain and a C-terminal dsRNA–binding domain (dsRBD). While E3L is thought to inhibit PKR activation by sequestering dsRNA activators and directly binding to the kinase, the role of the Zalpha domain in PKR inhibition is unclear. We recently showed that the E3L Zalpha domain is required to suppress the growth-inhibitory properties associated with expression of human PKR in yeast, to inhibit PKR kinase activity in vitro, and to reverse the inhibitory effects of PKR on reporter gene expression in mammalian cells treated with dsRNA. Whereas previous studies revealed that the Z-DNA–binding activity of E3L is critical for viral pathogenesis, we identified point mutations in E3L that functionally uncoupled Z-DNA binding and PKR inhibition. Thus, our studies demonstrate that the variolavirus E3L Zalpha domain, but not its Z-DNA–binding activity, is required for PKR inhibition, and they support the notion that E3L contributes to viral pathogenesis by targeting PKR and other components of the cellular anti-viral defense pathway (1).
While the protein kinases GCN2, HRI, PKR, and PERK specifically phosphorylate eIF2alpha on Ser51 to regulate global and gene-specific mRNA translation, eIF2alpha is dephosphorylated by the broadly acting serine/threonine protein phosphatase 1 (PP1). In mammalian cells, the regulatory subunits GADD34 and CReP target PP1 to dephosphorylate eIF2alpha; however, as there are no homologs of these targeting subunits in yeast, it was unclear how GLC7, the functional homolog of PP1 in yeast, is recruited to dephosphorylate eIF2alpha. We recently showed that a novel N-terminal extension on yeast eIF2gamma binds to GLC7 and targets it to dephosphorylate eIF2alpha. Truncation or point mutations designed to eliminate the PP1–binding motif in eIF2gamma impaired eIF2alpha dephosphorylation both in vivo and in vitro. Moreover, replacement of the N terminus of eIF2gamma with the GLC7–binding domain from GAC1 or fusion of heterologous dimerization domains to eIF2gamma and GLC7, respectively, maintained eIF2alpha phosphorylation at basal levels. Taken together, our results indicate that, in contrast to the paradigm of distinct PP1 targeting or regulatory subunits, the unique N terminus of yeast eIF2gamma functions in cis to target GLC7 to dephosphorylate eIF2alpha (2).
Analysis of eIF2 binding to Met-tRNAiMet and the ribosome
The gamma subunit of eIF2 is a GTPase that, based on sequence and the structure of the archaeal homolog aIF2gamma, resembles the bacterial translation elongation factor EF-Tu. However, in contrast to EF-Tu, which binds to the A-site of the 70S ribosome, eIF2 binds Met-tRNAiMet to the P-site of the 40S subunit. To gain insights into how eIF2 binds to Met-tRNAiMet and then associates with the 40S ribosome, we used directed hydroxyl radical probing to identify eIF2 contacts within the 40S–eIF1–eIF1A–eIF2–GTP–Met-tRNAi–mRNA (48S) complex (3). We generated a cysteine-deficient version of Saccharomyces cerevisiae eIF2 and then introduced single Cys residues at predicted surface-exposed sites (based on the aIF2 structure) on eIF2alpha, eIF2beta, and eIF2gamma. The mutant proteins were purified from yeast and then derivatized with Fe(II)-BABE on the Cys residue. Following addition of hydrogen peroxide, hydroxyl radicals, formed in the vicinity of the ferrous iron, diffuse and cleave nucleic acid and protein backbones. Based on the structure of the EF-Tu ternary complex, we predicted that linkage of Fe(II)-BABE to domain III of eIF2gamma would result in cleavage of Met-tRNAiMet in the T-stem. Instead, cleavages were observed in the D-stem of Met-tRNAiMet and at the top of helix h44 of 18S rRNA. Based on the results of these and other cleavage experiments, and the fact that Met-tRNAiMet is bound to the P-site of the 40S subunit, we generated a model of the 48S complex in which domain III of eIF2gamma binds near 18S rRNA helix h44 and in which eIF2gamma contacts primarily the acceptor stem of Met-tRNAiMet. In this model of the eIF2 ternary complex, the Met-tRNAiMet is rotated nearly 180° relative to the position of the tRNA in the EF-Tu ternary complex. In support of the alternate models for the eIF2 and EF-Tu ternary complexes, we found that the EF-Tu-T394C mutation in domain III severely impaired Phe-tRNA binding, whereas the corresponding eIF2gamma-K507C mutation did not impair Met-tRNAiMet binding to eIF2. Thus, despite their structural similarity, eIF2 and EF-Tu bind to tRNA in substantially different manners, and we propose that the tRNA–binding domain III of EF-Tu has acquired a new function in eIF2gamma to bind to the ribosome (3).
Analysis of an eIF2gamma mutation that links intellectual disability to impaired translation initiation
While protein synthesis is known to play a critical role in learning and memory in diverse model systems, human intellectual disability syndromes have not been directly associated with alterations in protein synthesis. Moreover, the consequences of partial loss of eIF2gamma function or eIF2 integrity are unknown in mammals, including humans. Our collaborators in Israel and Germany identified a human X-chromosomal neurological disorder characterized by intellectual disability and microcephaly. Mapping studies identified the causative mutation as a single base change resulting in a missense mutation in eIF2gamma (encoded by EIF2S3). Biochemical studies of human cells overexpressing the eIF2gamma mutant and of yeast eIF2gamma with the analogous mutation revealed a defect in binding of the eIF2beta subunit to eIF2gamma. Consistent with this loss of eIF2 integrity, the mutation in yeast eIF2gamma impaired translation start codon selection and eIF2 function in vivo in a manner that was suppressed by overexpression of eIF2beta. The findings directly link intellectual disability with impaired translation initiation and provide a mechanistic basis for the human disease as a result of partial loss of eIF2 function (4).
Molecular analysis of the hypusine-containing protein eIF5A
The translation factor eIF5A, the only protein containing the unusual amino acid hypusine [Ne-(4-amino-2-hydroxybutyl)lysine], was originally identified based on its ability to stimulate a model assay for first peptide–bond synthesis. However, the precise cellular role of eIF5A was unknown. Using molecular-genetic and biochemical studies, we previously showed that eIF5A promotes translation elongation and that this activity is dependent on the hypusine modification. Given that eIF5A is a structural homolog of the bacterial protein EF-P, we proposed that eIF5A/EF-P is a universally conserved translation elongation factor.
Recently, it was shown that EF-P promotes translation of polyproline sequences by bacterial ribosomes. Using in vivo reporter assays, we showed that eIF5A in yeast stimulates the synthesis of proteins containing runs of three or more consecutive proline residues. Consistently, the expression of native yeast proteins containing homopolyproline sequences was impaired in eIF5A mutant strains. To support the in vivo findings, we used reconstituted yeast in vitro translation assays to monitor the impact of eIF5A on protein synthesis. We found that the synthesis of polyproline peptides, but not polyphenylalanine peptides, was critically dependent on addition of eIF5A. Toe-printing experiments revealed that addition of eIF5A relieved ribosomal stalling during translation of three consecutive proline residues in vitro. Consistent with the functions of eIF5A in promoting peptide bond synthesis, directed hydroxyl radical probing experiments localized eIF5A binding to near the E site of the ribosome with the hypusine residue of eIF5A adjacent to the acceptor stem of the P-site tRNA. Thus, we propose that eIF5A, like its bacterial ortholog EF-P, stimulates the peptidyl-transferase activity of the ribosome and facilitates the reactivity of poor substrates such as proline (5).
Publications
- Thakur M, Seo EJ, Dever TE. Variola virus E3L Z domain, but not its Z-DNA binding activity, is required for PKR inhibition. RNA 2014;20:214-227.
- Rojas M, Gingras AC, Dever TE. Protein phosphatase PP1/GLC7 interaction domain in yeast eIF2 bypasses targeting subunit requirement for eIF2 dephosphorylation. Proc Natl Acad Sci USA 2014;111:E1344-1353.
- Shin BS, Kim JR, Walker SE, Dong J, Lorsch JR, Dever TE. Initiation factor eIF2gamma promotes eIF2-GTP-Met-tRNAiMet ternary complex binding to the 40S ribosome. Nat Struct Mol Biol 2011;18:1227-1234.
- Borck G, Shin BS, Stiller B, Mimouni-Bloch A, Thiele H, Kim JR, Thakur M, Skinner C, Aschenbach L, Smirin-Yosef P, Har-Zahav A, Nürnberg G, Altmüller J, Frommolt P, Hofmann K, Konen O, Nürnberg P, Munnich A, Schwartz CE, Gothelf D, Colleaux L, Dever TE, Kubisch C, Basel-Vanagaite L. eIF2gamma mutation that disrupts eIF2 complex integrity links intellectual disability to impaired translation initiation. Mol Cell 2012;48:641-646.
- Gutierrez E, Shin BS, Woolstenhulme CJ, Kim JR, Saini P, Buskirk AR, Dever TE. eIF5A promotes translation of polyproline motifs. Mol Cell 2013;51:35-45.
Collaborators
- Guntram Borck, MD PhD, Universität Ulm, Ulm, Germany
- Allen R. Buskirk, PhD, Brigham Young University, Provo, UT
- Anne-Claude Gingras, PhD, Samuel Lunenfeld Research Institute, Mount Sinai Hospital, and University of Toronto, Toronto, Canada
- Rachel Green, PhD, The Johns Hopkins University, Baltimore, MD
- Jon R. Lorsch, PhD, The Johns Hopkins University, Baltimore, MD
- Frank Sicheri, PhD, Samuel Lunenfeld Research Institute, Mount Sinai Hospital, and University of Toronto, Toronto, Canada
Contact
For more information, email tdever@box-t.nih.gov or visit spb.nichd.nih.gov