You are here: Home > Section on Molecular Morphogenesis
Thyroid Hormone Regulation of Vertebrate Postembryonic Development
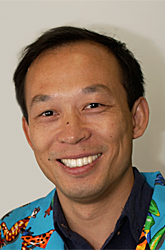
- Yun-Bo Shi, PhD, Head, Section on Molecular Morphogenesis
- Liezhen Fu, PhD, Staff Scientist
- Thomas Miller, PhD, Postdoctoral Intramural Research Training Award Fellow
- Morihiro Okada, PhD, Visiting Fellow
- Julia Rodiger, PhD, Visiting Fellow
- Luan Wen, PhD, Visiting Fellow
- Nga Luu, MS, Biologist
- Dan Su, MD, Adjunct Scientist
The laboratory investigates the molecular mechanisms of thyroid hormone (TH) function during postembryonic development. The main model is the metamorphosis of Xenopus laevis and X. tropicalis, two highly related species that offer unique but complementary advantages. The control of this developmental process by TH offers a paradigm to study gene function in postembryonic organ development. During metamorphosis, different organs undergo vastly different changes. Some, like the tail, undergo complete resorption, while others, such as the limb, are developed de novo. The majority of larval organs persist through metamorphosis but are dramatically remodeled to function in a frog. For example, tadpole intestine is a simple tubular structure consisting primarily of a single layer of larval epithelial cells. During metamorphosis, it is transformed into an organ with a multiply folded adult epithelium surrounded by elaborate connective tissue and muscles by a process involving specific larval epithelial cell death and de novo development of the adult epithelial stem cells, followed by their proliferation and differentiation. The wealth of knowledge from past research and the ability to manipulate amphibian metamorphosis, both in vivo by using genetic approaches or hormone treatment of whole animals and in vitro in organ cultures, offer an excellent opportunity to (i) study the developmental function of TH receptors (TRs) and the underlying mechanisms in vivo and (ii) identify and functionally characterize genes critical for postembryonic organ development in vertebrates. Our studies revealed likely conserved mechanisms in adult intestinal stem cell development in vertebrates and prompted us to adapt a mouse model to complement the amphibian system for gene knockout studies.
Unliganded TRα controls developmental timing in Xenopus tropicalis.
Earlier studies showed that, of the two TR genes (TRα and TRβ), TRα expression appears earlier during development than TH synthesis and secretion into the plasma. This and the ability of TRs to regulate gene expression both in the presence and absence of TH pointed to a role of unliganded TR during vertebrate development. However, the study of the role of unliganded TR during development in mammals is problematic because it is difficult to manipulate uterus-enclosed, late-stage embryos. Amphibian development, on the other hand, offers an excellent opportunity to address this question. We designed TALENs (transcriptional activator–like effector nucleases) to mutate the TRα gene in X. tropicalis. We showed that knockdown of TRα enhances tadpole growth in premetamorphic tadpoles, in part owing to elevated growth hormone gene expression. More importantly, the knockdown also accelerates animal development, with knockdown animals initiating metamorphosis at a younger age and with a smaller body size than control animals. However, such tadpoles are resistant to exogenous TH and exhibit delayed natural metamorphosis. Thus, our studies not only directly demonstrated a critical role of endogenous TRα in mediating the metamorphic effect of TH but also revealed novel functions for unliganded TRα during early development, that is, in regulating both tadpole growth rate and the timing of metamorphosis.
TH-induced histone methyltransferase Dot1L is required for postembryonic development but dispensable for Xenopus embryogenesis.
In our study of gene regulation by TR during metamorphosis, we discovered that the methylation levels of histone H3K79 at TH target genes were upregulated during natural and TH–induced metamorphosis. Interestingly, the histone methyltransferase Dot1L is the only known enzyme capable of methylating histone H3K79, suggesting that Dot1L may function as a TR coactivator. In addition, we showed that Dot1L is directly activated by TH via TR at the transcription level. To investigate the role of Dot1L during metamorphosis, we generated a Dot1L–specific TALEN nuclease to knockdown endogenous dot1L in X. tropicalis, a diploid species closely related to the well-known developmental model X. laevis, a pseudotetraploid amphibian. We demonstrated that the TALEN was extremely efficient in mutating dot1L when expressed in fertilized eggs, creating essentially dot1L knockout embryos with little H3K79 methylation (1). Importantly, we observed that dot1L knockdown had no apparent effect on embryogenesis, given that normal, feeding tadpoles were formed. By contrast, dot1L knockdown severely retarded growth of the tadpoles and led to tadpole lethality prior to metamorphosis. The findings and the lack of maternal dot1L expression suggest that Dot1L and methylation of H3K79 are dispensable for embryogenesis but essential for tadpole growth and development prior to metamorphosis into a frog. Our findings further reveal interesting similarities and differences between Xenopus and mouse development and suggest the existence of two separate phases of vertebrate development with distinct requirements for epigenetic modifications.
Differential regulation of two histidine ammonia-lyase genes during Xenopus development points to distinct functions during TH–induced formation of adult stem cells.
We showed earlier that de novo formation of adult intestinal stem cells requires TH signaling in both the larval epithelium (Ep) and non-epithelial tissues (non-Ep). To understand the underlying molecular mechanisms, it is necessary to determine the genes and signaling pathways involved in this process. Thus, we profiled the gene expression programs in Ep and non-Ep tissues in X. laevis intestine to systematically determine changes underlying the cell-autonomous and cell-cell interaction–dependent processes that are required for stem cell formation (2). One gene thus identified is the histidine ammonia-lyase (HAL) gene, which encodes an enzyme known as histidase or histidinase. We showed that there are two duplicated HAL genes, hal1 and hal2, in both X. laevis and X. tropicalis, the latter a closely related but diploid species. Interestingly, only hal2 is highly upregulated by TH and appears to be specifically expressed in the adult intestinal progenitor/stem cells, while hal1 is not expressed in the intestine during metamorphosis. Furthermore, when analyzed in whole animals, hal1 appears to be expressed only during embryogenesis but not metamorphosis, while the opposite appears to be true for hal2. Our results suggest that the duplicated HAL genes have distinct functions, with hal2 likely involved in the formation and/or proliferation of the adult stem cells during metamorphosis.
Direct activation of sox3 gene transcription by TH in the developing adult intestinal stem cell during Xenopus metamorphosis
We also discovered from our microarray studies that the transcription factor Sox3, well known for its involvement in neural development, was upregulated in the intestinal epithelium during metamorphosis (2). We then showed that Sox3 is highly and specifically expressed in the developing adult intestinal progenitor/stem cells. We further demonstrated that sox3 is a direct target gene of TH, given that its induction by TH does not depend on new protein synthesis, suggesting that TH activates the promoter directly via TH receptor (3). Thus, TH activation of Sox3 is one of the earliest changes in the epithelium and, in turn, Sox3 may facilitate de-differentiation of larval epithelial cells into adult stem cells.
Global expression profiling reveals genetic programs underlying the developmental divergence between mouse and human embryogenesis.
In a collaborative study, we analyzed developmental gene expression profiles in mouse. Mouse has served as an excellent model for studying human development and diseases. Over the past few decades, advances in transgenic and knockout studies in mouse have dramatically strengthened the use of this model and significantly improved our understanding of gene function during development. However, little is known about the gene-regulatory networks governing mouse organogenesis. Importantly, mouse and human development diverge during organogenesis. For instance, the mouse embryo is born around the end of organogenesis while in the human the subsequent fetal period of ongoing growth and maturation of most organs spans more than two-thirds of human embryogenesis. To investigate underlying molecular mechanisms, we carried out a detailed analysis of global gene expression profiles from egg to the end of organogenesis in mouse. Our studies revealed distinct temporal regulation patterns for genes belonging to different functional (Gene Ontology or GO) categories that support their roles during organogenesis. More importantly, comparative analyses identified both conserved and divergent gene-regulation programs in mouse and human organogenesis, with the latter likely responsible for the developmental divergence between the two species, and further suggest a novel developmental strategy during vertebrate evolution. Based on our earlier observation that gene function in a given process tends to be developmentally co-regulated during organogenesis, our microarray data should help identify genes associated with mouse development and/or infer the developmental functions of unknown genes. Our study might also be useful for investigating the molecular basis of vertebrate evolution.
Slc7a5, the catalytic subunit of the system L1 amino acid/thyroid hormone transporter (LAT1), facilitates nutrient signaling in mouse skeletal muscle.
To regulate cellular processes, TH has to be actively transported into cells, a process that is mediated by several different types of transporters. One of our previously identified TH–response genes in the intestine, Slc7a5, encodes the light chain of a heterodimeric system L–type of TH transporter (LAT1), which also transports several amino acids. Interestingly, Lat1 is highly upregulated at the climax of metamorphosis in the tadpole intestine, coinciding with the formation and rapid proliferation of adult intestinal stem cells. We recently found that LAT1 was also highly expressed in the mouse intestine during the neonatal period when the mouse intestine matures into the adult form, a process that appears also to involve TH–dependent formation and/proliferation of adult intestinal stem cells. In a further collaborative study, we generated a mouse line with the Slc7a5 gene floxed, which allows conditional knockout of Slc7a5 upon expression of the Cre recombinase (4). When we crossed floxed Slc7a5 mice with mice expressing Cre driven by a global promoter, we obtained global Slc7a5 heterozygous knockout (Slc7a5+/−) animals; failure to produce homozygous Slc7a5 animals indicates that the global knockout of Slc7a5 is embryonically lethal. Slc7a5 mRNA expression and functional LAT1 transport activity were reduced in the Slc7a5+/− animals, although no growth phenotype was detected. Muscle-specific (MCK Cre-mediated) Slc7a5 knockout mice were established and used to study the role of SLC7A5 in the intracellular delivery of large neutral amino acids (LNAA) such as leucine, which are required for full activation of the mTOR-S6K signaling pathway, promoting protein synthesis and cell growth. Activation of muscle mTOR-S6K (Thr389 phosphorylation) by intraperitoneal leucine injection was blunted in homozygous MCK Cre-Slc7a5-Flox mice compared with wild-type animals. As dietary protein content was reduced from 30 to 10%, Leu and Ile concentrations in gastrocnemius muscle fell by about 40%. The changes were associated with a greater than 50% reduction in S6K Thr389 phosphorylation in Slc7a5-knockout muscle, indicating reduced mTOR-S6K pathway activation, despite no significant differences in lean tissue mass between groups on the same diet. MCK Cre-Slc7a5-Flox mice on 30% protein diet exhibited mild insulin resistance (e.g., reduced glucose clearance, larger gonadal adipose depots) compared with control animals. Thus, SLC7A5 modulates LNAA–dependent muscle mTOR-S6K signaling in mice, although it appears non-essential for maintenance of normal muscle mass. In addition, we had shown earlier, in another collaboration, that LAT1 is critical for antigen receptor–mediated metabolic re-programming (5), a process essential for T cell differentiation, suggesting LAT1 plays diverse roles in several tissues/organs.
Publications
- Wen L, Fu L, Guo X, Chen Y, Shi Y-B. Histone methyltransferase Dot1L plays a role in postembryonic development in Xenopus tropicalis. FASEB J 2014;E-pub ahead of print.
- Sun G, Heimeier RA, Fu L, Hasebe T, Das B, Ishizuya-Oka A, Shi Y-B. Expression profiling of intestinal tissues implicates tissue-specific genes and pathways essential for thyroid hormone-induced adult stem cell development. Endocrinology 2013;154:4396–4407.
- Sun G, Fu L, Wen L, Shi Y-B. Activation of Sox3 gene by thyroid hormone in the developing adult intestinal stem cell during Xenopus metamorphosis. Endocrinology 2014;E-pub ahead of print.
- Poncet N, Mitchell FE, Ibrahim AFM, McGuire VA, English G, Arthur SC, Shi Y-B, Taylor PM. The catalytic subunit of the System L1 amino acid transporter (Slc7a5) facilitates nutrient signaling in mouse skeletal muscle. PLoS One 2014;9:e89547,1-14.
- Sinclair LV, Rolf J, Emslie E, Shi Y-B, Taylor PM, Cantrell DA. Antigen receptor control of amino acid transport coordinates the metabolic re-programming that is essential for T cell differentiation. Nat Immunol 2013;14:500-508.
Collaborators
- Doreen A. Cantrell, PhD, University of Dundee, Dundee, UK
- Yonglong Chen, PhD, Guangzhou Institute of Biomedicine and Health, Guangzhou, China
- Atsuko Ishizuya-Oka, PhD, Nippon Medical School, Tokyo, Japan
- Wenxin Li, PhD, Wuhan University, Wuhan, China
- Peter Taylor, PhD, University of Dundee, Dundee, UK
Contact
For more information, email shi@helix.nih.gov or visit smm.nichd.nih.gov.