You are here: Home > Section on Endocrine Physiology
Neuroendocrinology of Stress
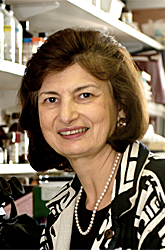
- Greti Aguilera, MD, Head, Section on Endocrine Physiology
- Jun Chen, PhD, Postdoctoral Fellow
- Ying Liu, MD, Research Associate
The goal of the laboratory is to understand the neuroendocrine mechanisms underlying the stress response, with emphasis on the regulation of the hypothalamic pituitary adrenal (HPA). Not only during early development but also during adult life, the ability of the organism to adapt to acute and chronic stress situations is determined by genetic constitution and life experiences. The organism’s degree of adaptability may lead to long-term consequences for the responsiveness of the HPA axis, with altered expression of hypothalamic corticotrophin releasing hormone (CRH) and circulating levels of glucocorticoids—hormones implicated in the pathogenesis of several psychiatric and metabolic disorders. Our laboratory studies the mechanisms of positive and negative regulation of expression of the hypothalamic hormones CRH and vasopressin (VP) and their receptors under different stress situations and the impact of such stress situations on HPA axis regulation. The influence of life experiences, especially during early development, on neuroendocrine regulation and the expression of genes involved in the stress response are important aspects of our research program. Elucidation of the effects of life experiences on the stress response and its regulation is critical for understanding the mechanisms leading to HPA axis dysregulation and for developing diagnostic, preventive, and therapeutic tools for stress-related disorders.
Neuroprotective actions of VP
VP that is secreted within the brain modulates neuronal function by acting as a neurotransmitter. We previously showed that expression of VP in parvocellular neurons of the hypothalamic paraventricular nucleus increases markedly during prolonged stress, but that VP plays a relatively minor role in the regulation of the hypothalamic pituitary adrenal axis under chronic conditions. These observations led to the search for new roles of vasopressin during stress adaptation.
Recent studies from this laboratory using the neuronal cell line H32, which expresses endogenous V1 VP receptors, showed that activation of endogenous V1 VP receptors prevents serum deprivation–induced apoptosis. The mechanism by which VP prevents apoptosis involves phosphorylation-inactivation of the pro-apoptotic protein Bad, with consequent decreases in cytosolic cytochome c and reduction in caspase-3 activation. These actions of VP are largely mediated by mitogen-activated protein (MAP) kinase and extracellular signal–regulated kinases (ERK) but also involve other signaling pathways.
Studies during the past year examined the role of protein kinases C (PKC) and B (PKB) in mediating VP-induced anti-apoptosis in H32 cells. Serum deprivation increased PKCdelta but not PKCalpha and PKCbeta activity. Inhibition of PKCdelta prevented caspase-3 activation, indicating that PKCdelta mediates the proapoptotic actions of serum deprivation. On the other hand, VP increased PKCalpha and PKCbeta without affecting the increases in PKCdelta activity. Simultaneous inhibition of PKCalpha and PKCbeta and MAP kinase abolished VP-induced Bad phosphorylation but only partially prevented caspase-3 inhibition. Complete abolition of the protective effect of VP on serum deprivation–induced caspase 3 activity required additional blockade of PI3K/protein kinase B (Akt). The data demonstrate that VP exerts its anti-apoptotic effects through multiple pathways; while PKCalpha and PKCbeta, together with ERK/MAP kinase, activation mediates Bad phosphorylation (inactivation), the full protective action of VP requires additional activation of PKB (PI3K/Akt) pathway.
The studies in H32 cells demonstrate that VP exerts anti-apoptotic effects in a neuronal cell line and suggest that VP has neuroprotective properties. This hypothesis was tested in primary cultures of hippocampal neurons by examining the ability of VP to prevent nutrient deprivation–induced or glutamate-induced apoptosis and the signaling pathways involved in this effect. Deprivation of trophic factors (by changing the defined media supplement by 0.1%BSA) for 24 hours or exposure to glutamate (3 M) caused a significant decrease in neuronal cell viability and increases in Tdt-mediated dUTP nick-end labeling (TUNEL) staining and caspase-3 activity, consistent with apoptotic cell death. The effects of trophic factor deprivation or glutamate were significantly reduced by addition of 10nM VP, suggesting that VP exerts anti-apoptotic effects in neurons. This was confirmed by the ability of VP to prevent trophic factor deprivation–induced or glutamate-induced TUNEL staining of neurons. The protective effect of VP was completely blocked by the V1 receptor antagonist (Phenylac1,D-Tyr(Et)2,Lys6,Arg8,des-Gly9)-Vasopressin, indicating that the effect is mediated via V1 VP receptors. The anti-apoptotic effect of VP in neurons involves MAP kinase, ERK, and PI3 kinase/Akt signaling pathways. This was shown by the ability of the selective MAPK inhibitor U0126 or the PI3 kinase inhibitor LY294002 to reduce the inhibitory action of VP on caspase-3 activity and TUNEL staining of neurons in cultures exposed to nutrient deprivation or glutamate. Western blot analysis revealed biphasic increases in phosphorylation/activation of ERK and Akt during incubation with VP. These data show that VP has anti-apoptotic actions in neurons, an effect that is partially mediated by the MAPK signaling pathway. The study supports the hypothesis that VP released within the brain can act as a neuroprotective agent in the brain.
Regulation of hypothalamic CRH expression
Appropriate responses of the HPA axis to stress, with adequate control of adrenal glucocorticoid secretion, are essential for homeostasis. Studies of this laboratory have made important contributions to our understanding the role of the interaction between CRH and VP in the regulation of pituitary ACTH and of the expression of these peptides in the paraventricular nucleus (PVN) during stress and other alterations of the HPA axis. Both peptides co-expressed in the same parvocellular neuron of thePVN are differentially regulated during stress or exposure to glucocorticoids. CRH coordinates behavioral, autonomic and hormonal responses to stress and is the main regulator of ACTH secretion in acute and chronic conditions. Following CRH release, activation of CRH transcription is required to restore mRNA and peptide levels, but termination of the response is essential to prevent the pathology associated with chronic elevation of CRH and glucocorticoid production. This laboratory has advanced our understanding of the mechanisms controlling negative and positive transcriptional regulation of CRH. CRH transcription is under positive control by cAMP/phospho-CREB signaling and negatively regulated by glucocorticoid feedback. Our research has demonstrated that, in addition to glucocorticoids, intracellular feedback mechanisms in the CRH neuron, involving induction of the repressor form of cAMP response element modulator (CREM)—theinducible cyclic AMP early repressor (ICER). Recruitment of ICER by the CRH promoter limits CRH transcription by competing with the positive regulator phospho-CREB. Rapid repression of CRH transcription following stress-induced activation is likely to contribute to limiting the stress response and to preventing disorders associated with excessive CRH production.
CRH transcription requires the CREB co-activator TORC
We have solid evidence that cAMP/phospho–CREB signaling is essential but not sufficient to activate CRH transcription. This finding strongly suggested that transcriptional activation requires a co-activator. Studies in other CREB-regulated genes, such as those encoding glyconeogenic enzymes, and steroidogenic proteins such as StAR protein and side chain cleavage enzyme, have shown that initiation of transcription requires nuclear tanslocation of the CREB co-activator called transducer of regulated CREB activity (TORC). Three subtypes of TORC have been identified: TORC1, TORC2, and TORC3. In basal conditions, TORC is inactive and in its phosphorylated state remains in the cytoplasm bound to the scaffolding protein 14-3-3. Activation of the cyclic AMP/PKA pathway inhibits the kinases responsible for TORC phosphorylation—AMP dependent kinase (AMPK) and salt inducible kinase (SIK)—thus allowing dephosphorylation and nuclear translocation. The hypothesis that TORC is involved in the regulation of CRH transcription was tested using the hypothalamic cell line 4B. This cell line does not express endogenous CRH but provides a good system for studying CRH transcription using reporter gene assays. Quantitative real time PCR indicated that all three TORC subtypes are present in 4B cells, with TORC2 being the most abundantly expressed (TORC2 levels are 5-fold greater than TORC1 and 15-fold greater than TORC3). Western blot analysis of cytoplasmic and nuclear proteins revealed rapid and transient nuclear translocation of TORC2 and TORC3, and to a minor extent TORC1, by forskolin in a dose-dependent manner. In contrast, the phorbol ester PMA had no effect on nuclear TORC levels and caused a delay in migration in the cytoplasm, suggesting hyper-phosphorylation. In reporter gene assays, co-transfection of expression vectors for TORC1 or TORC2 increased basal CRH promoter activity and potentiated the stimulatory effect of forskolin. The phorbol ester PMA had no significant effect on CRH promoter activity, with or without TORC over-expression. Silencing-RNA knock out of each endogenous TORC subtype partially inhibited forskolin-stimulated CRH promoter activity, while simultaneous knockout of TORC2 and TORC3 was sufficient for complete inhibition. Co-immunoprecipitation and chromatin immunoprecipitation experiments revealed association of CREB and TORC in the nucleus, and recruitment of TORC2 by the CRH promoter, following 30-minute incubation with forskolin. The data demonstrate that, acting as a CREB co-activator, TORC2 is required for transcriptional activation of the CRH promoter in the hypothalamic cell line 4B. In addition, cytoplasmic retention of TORC during PMA treatment is likely to explain the failure of phorbolesters to activate CRH transcription in spite of efficiently phosphorylating CREB.
The physiological relevance of these findings was studied in primary cultures of hypothalamic neurons in vitro and hypothalamic tissue from control and stressed rats. Western blot analysis of hypothalamic proteins and antibodies to TORC1, TORC2, and TORC3 revealed the presence of TORC1 and TORC2 in cytoplasmic and nuclear fractions. A 30-minute restraint stress increased TORC 2 in the nuclear fractions, which was followed by a decrease to basal by two hours of restraint. There were no significant changes in TORC1 levels in cytoplasmic of nuclear proteins at either duration of stress. Similar results were observed in hypothalamic neuronal cultures following a 20-minute incubation with forskolin, with only TORC 2 translocating to the nucleus. Immunohistochemical studies using TORC2 antibody in rat hypothalamic tissue, performed in collaboration with Valery Grinevich, revealed specific staining in the parvocellular and magnocellular areas of the PVN. Staining was mostly cytoplasmic in controls and after four hours of restraint; after 30 minutes of restraint, there was a significant increase in nuclear staining in the parvocellular but not magnocellular region. Double fluorescent immunostaining and confocal microscopy showed that 61 (3.5%) of CRH immunoreactive cells also stained for TORC2. The demonstration of the presence of TORC 2 in CRH neurons and its transient translocation to the nucleus during stress supports the involvement of this TORC subtype in CRH regulation. Our current research focuses on the interactions of TORC with the CRH promoter and on the importance of the co-activator TORC during physiological regulation of CRH transcription in vivo.
Early life stress and CRH transcription
During the past year, we placed considerable emphasis on studying the long-term consequences of early life stress on the function of the HPA axis. It is well recognized that stress exposure during early development causes long-lasting alterations in behavior and HPA axis activity, including increased levels of CRH mRNA in the PVN. The aim of this study was to test the hypothesis that early life stress causes epigenetic changes in the CRH promoter leading to increased CRH transcription. ACTH and corticosterone, and CRH primary transcript or hnRNA levels (as an index of CRH transcription) were evaluated in groups of 8-week old female and male rats, which had been subjected to maternal deprivation (MD) between days 2 and 10 post-birth, either in basal conditions, or following 30 or 60 min restraint stress. Groups of control and MD rats were also used for methylation analysis of the CRH promoter in the PVN and amygdala. Adrenal weight, basal levels of plasma corticosterone, and hypothalamic CRH hnRNA were elevated in MD females but not in males. However, plasma corticosterone and CRH hnRNA responses to acute restraint stress were elevated in MD of both sexes. DNA methylation analysis of the CRH promoter revealed, in both sexes, a lower percent of methylation specifically in two CpGs located immediately preceding (CpG1) and inside (CpG2) the cAMP-responsive element (CRE) at−230,in the PVN and amygdala. This CRE has been shown to be an absolute requirement for activation of the CRH promoter. In contrast to the PVN, the percentage of methylation of CpG1 and CpG2 in the amygdala were identical in control in rats subjected to maternal deprivation. These findings demonstrate that HPA axis hypersensitivity caused by neonatal stress causes long-lasting enhanced CRH transcriptional activity in the PVN of both sexes. Hypomethylation of the−230 CRE in the CRH promoter is likely to serve as a mechanism for the increased transcriptional responses to stress observed in maternal deprivation in rats. Our current efforts are directed at elucidating the functional consequences of hypomethylation of CpG1 and CpG2. Specifically, we will study the capacity of the CRH promoter CRE to bind to the transcription factor phospho-CREB and to the co-activator TORC2, as well as the association of acetylated histones and methyl DNA binding protein to the CRH promoter.
Publications
- Liu Y, Kamitakahara A, Kim AJ, Aguilera G. Cyclic AMP responsive element binding protein phosphorylation is required but not sufficient for activation of CRH transcription. Endocrinology 2008 49:3512-3520.
- Chen J, Volpi S, Aguilera G. Anti-apoptotic actions of vasopressin in H32 neuronal cells involve MAP kinase transactivation and Bad phosphorylation. Exp Neurol 2008 211:529-538.
- Chen J, Liu Y, Aguilera G. Antiapoptotic effects of vasopressin in the neuronal cell line H32 involve protein kinase C alpha and beta and protein kinase B. J Neurochem 2009 110:1310-1320.
- Liu Y, Aguilera G. Cyclic AMP inducible early repressor (ICER) mediates the termination of corticotropin releasing hormone transcription in hypothalamic neurons. Mol Cell Neurobiol 2009 [E-pub ahead of print].
- Blume A, Torner L, Liu Y, Subburaju S, Aguilera G, Neumann ID. Prolactin activates MAP kinase signaling and corticotropin releasing hormone (CRH) transcription in rat hypothalamic neurons. Endocrinology 2009 150:1841-1849.
Collaborator
- Valery Grinevich, MD, PhD, DSc, Max Plank Institute, Heidelberg, Germany
Contact
For more information, email Greti_Aguilera@nih.gov.