You are here: Home > Section on Hormonal Regulation
Peptide Hormone Receptors and Signal Transduction
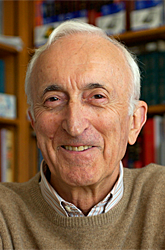
- Kevin J. Catt, MD, PhD, Head, Section on Hormonal Regulation
- Albert Baukal, Research Assistant
- Hung-Dar Chen, PhD, Adjunct Investigator
- Hao Feng, MD, PhD, Visiting Fellow
- Lazar Krsmanovic, PhD, Staff Scientist
- Po Ki Leung, PhD, Visiting Fellow
- Anli Tong, MD, PhD, Volunteer
This program investigates the mechanisms of action of regulatory peptide hormones in specific endocrine systems, mainly those that mediate reproductive hormone secretion (GnRH) and cardiovascular function (Angiotensin II). The research includes analysis of two specific G protein–coupled receptors (GPCRs) that bind to GnRH and the angiotensin II octapeptide. The GnRH receptor mediates pituitary function and gonadotropin secretion from the anterior pituitary gland and controls the molecular and cellular systems that regulate the secretion and actions of the gonadotropin-releasing hormone (GnRH). Our studies address the structure-function properties and signaling mechanisms of the GnRH receptor, which we found to be expressed in hypothalamic GnRH neurons as well as in the pituitary gonadotroph cells. It is also a unique G protein–coupled receptor that lacks a C-terminal cytoplasmic domain and has other unusual structural features, including a highly basic first intracellular loop. The GnRH receptor is primarily coupled to the phosphoinositide/calcium signaling pathway through Gq/11, and also activates the adenylyl cyclase system through Gs. The first intracellular loop of the GnRH receptor, expressed in COS-7 cells, contains at least four amino acids that are necessary for its coupling to Gs and activation of adenylyl cyclase, but not for coupling to Gq and phospholipase C activation. Thus, Gs signaling depends on the first intracellular loop of the GnRH receptor and Gq signaling to phospholipase C on interactions with the second and third intracellular loops. The positively charged residues are essential for interactions between the first loop and Gs, and for the GnRH receptor–activated cyclic AMP response.
Regulation of GnRH secretion in vivo is also influenced by the cholinergic system as well as the aminergic and peptidergic systems. GnRH neurons and their immortalized counterparts (GT1 cells, created by Pamela Mellon of UCSD) produce acetylcholine, and both cell types are regulated by cholinergic receptors. The GnRH release profiles of cells stimulated with acetylcholine were found to result from activation of both nicotine and muscarinic receptors, which respectively have stimulatory and predominantly inhibitory effects on neurosecretion. Agonist-induced changes in Gq and Gi α-subunits in cell membranes during muscarinic receptor stimulation are correlated with activation of phospholipase C and adenylyl cyclase, respectively. The ability of acetylcholine to differentially modulate GnRH release from hypothalamic neurons through nicotinic and both M1 and M2 receptors indicates the significance of cholinergic inputs in the control of neurosecretion.
We performed further studies on the pulsatile release of GnRH in vitro on GT1-7 cells and cultured fetal hypothalamic cells. Such cultures, as well as hypothalamic tissue from adult rats, express GnRH receptors, as evidenced by the presence of high-affinity GnRH binding sites and GnRH receptor transcripts. Furthermore, we found that individual GnRH neurons co-express both the GnRH peptide and its GnRH receptors, as revealed by double immunostaining of hypothalamic cultures. In static cultures of hypothalamic neurons and GT1-7 cells, treatment with GnRH receptor antagonists caused a prominent increase in GnRH release. In perifused hypothalamic cells and GT1-7 cells, GnRH antagonist analogs abolished the basal mode of pulsatile secretion and caused a sustained and progressive increase in GnRH release.
In contrast, a GnRH receptor agonist reduced the frequency and increased the amplitude of pulsatile GnRH release, as previously observed in GT1-7 cells. Such findings demonstrate that functional GnRH receptors are expressed in cultured hypothalamic GnRH neurons, as formerly shown for the GT1-7 line of immortalized GnRH neurons. In addition, the activation of such autoreceptors is required for pulsatile GnRH release from the hypothalamic GnRH neurons, and is essential for pulsatile GnRH release from the hypothalamic GnRH neuronal network in vitro. The effects of GnRH agonist and antagonist analogs on neuropeptide release are consistent with the operation of an ultrashort-loop autocrine feedback mechanism exerting both positive and negative actions that contribute to the integrated control of GnRH secretion from the hypothalamus.
Functional characterization of GnRH receptors in immortalized GnRH neurons
The G protein–coupled receptor (GPCR) systems have multi-modular arrangements and provide cells with several possibilities to transduce incoming signals. A typical cell can express several GPCR genes, several combinations of G protein subunits, and multiple isoforms of effector molecules that can be activated by each type of G protein. The differential expression of these proteins allows modulation of signals at different levels, resulting in signaling that is characteristic of a specific cell type. The current understanding is that protein-protein interactions determine the mechanisms of interaction between the G protein and a particular GPCR. However, several observations in specific cells and tissues have shown that different receptors coupling to the same G protein in a single cell can elicit differential biochemical or cellular responses. The endogenous GnRH-Rs expressed in native and immortalized (GT1-7) GnRH neurons activate at least three G proteins, as indicated by the agonist-induced release of their specific α-subunits from the plasma membrane. Such coupling to a diverse array of G proteins provides GnRH neurons with several potential signaling pathways to transduce incoming signals. The differential expression of these various proteins allows modulation of signals at many levels, resulting in messages that are optimal for specific cell functions.
We monitored cellular distribution, receptor-receptor interactions, and signaling pathways of the murine GnRH-R tagged with Renilla luciferase (Rluc) and/or green fluorescent protein (GFP) in GT1-7 GnRH neurons. GFP-tagged GnRH-R was confined to a thin rim of cytosol at the plasma membrane, neuronal processes, and apparent synaptic junctions. GnRH stimulation caused redistribution of GnRH-R-GFP, with movement in close proximity to their bipolar extensions and increased intensity in synaptic connections. BRET2 assay revealed dose-dependent, GnRH-induced interaction between GFP-tagged GnRH-R and Rluc-tagged Gαq, Gαs, and Gαi. Firing of spontaneous action potentials (APs), calcium signaling, and pulsatile GnRH secretion were comparable to those of intact GT1-7 neurons. These findings indicate that specific subcellular localization, agonist-induced redistribution, homologous oligomerization, and coupling to the multiple G proteins of the GnRH-R receptor modulate electrical activity, second messengers, and neurosecretion of the hypothalamic GnRH neurons.
Roles of tyrosine322 and tyrosine324 in GnRH receptor function
With several unique structural features, the GnRH receptor is an anomalous member of the GPCR superfamily. These include (1) the absence of a cytoplasmic C-terminal tail; (2) the replacement of Tyr by Ser in the conserved DRY motif located at the junction of transmembrane domain (TMD) III and the 2i loop; (3) interchange of conserved Asp and Asn residues in TMDs II and VII; and (4) a relatively long and highly basic 1i loop. The Asn-Pro-any amino acid-Tyr (NPXY) sequence, where X represents an aliphatic amino acid, mediates interactions with intracellular effector molecules. The NPXY motif is present in TM VII of the GnRH-R and is conserved among sevenTM receptors.
We analyzed GnRH receptor binding properties and cAMP signaling in GT1-7 cells expressing the endogenous GnRH-R and in COS-7 cells transfected with the mouse GnRH-R. Agonist stimulation of the wild-type GnRH-R, expressed in COS-7 cells using a pGFP2-N vector, caused a monotonic dose-dependent increase in cAMP production. Deletion of Tyr322, or its change to Ser, increased GnRH binding capacity of the mutant receptor 3–5 fold. In such receptors, GnRH-induced cAMP production was abolished, indicating the absence of Gs activation. GnRH-induced phosphorylation of mitogen-activated protein kinases (MAPKs) was also abolished in the mutant receptor, consistent with impairment of the protein kinase C (PKC)–dependent signaling pathway. GnRH-R binding activity was abolished when Tyr322 was substituted with Phe. Substitution of Tyr324 of the mouse GnRH-R with non-aromatic hydroxyl groupcontaining Ser caused loss of agonist binding. In contrast, when Tyr324 was replaced with Phe, the mutant receptor retained high binding capacity and cAMP signaling comparable to that of the wild-type GnRH-R. These findings demonstrate that both Tyr322 and Tyr324 of the mouse GnRH receptor are crucial residues that determine the receptor's agonist-binding and signaling properties.
GnRH-induced modulation of after-hyperpolarizing current in GnRH neurons
In many central nervous system neurons, action potentials (APs) are followed by prolonged afterhyperpolarization (AHP) of the membrane potential, which controls excitability and firing pattern. Two classes of AHPs and the underlying Ca2+-activated currents can be distinguished by their time course and pharmacological properties. IAHP (inward after-hyperpolarization potential) underlies part of the medium AHP (mAHP) following single or repetitive APs, is sensitive to the bee venom toxin apamin, and exhibits rapid rise and decay (time constants 100 ms).
Endogenous GnRH receptors expressed in native and immortalized GnRH neurons initiate diverse signaling pathways by coupling to multiple G proteins. Such coupling is time- and dose-dependent, and can switch between Gq, Gs, and Gi/o, in GnRH-stimulated GT1-7 neurons. These findings suggest that an agonist concentration-dependent switch in coupling of the GnRH-R between specific G proteins modulates neuronal Ca2+ signaling via Gs-cAMP–stimulatory and Gi-cAMP–inhibitory mechanisms. Activation of Gi may also inhibit GnRH neuronal function and episodic secretion by regulating membrane ion currents, possibly through activation of G protein–regulated inwardly rectifying potassium channels (GIRKs). Such channels are important for maintaining the resting potential and excitability of neurons. Accordingly, it is necessary to clarify the functional activities of these channels associated with regulation of the GnRH neuron, and its spontaneous electrical activity and episodic neuropeptide secretion.
Action potentials and the associated Ca2+ influx are followed by slow afterhyperpolarizations (sAHPs) caused by a voltage-insensitive, Ca2+-dependent K+ current. Slow AHPs are common in mammalian neurons and are present in both peripheral and central nervous systems. The firing of individual and/or bursts of APs in spontaneously active GnRH neurons is followed by hyperpolarization that lasts from several milliseconds (ms) to several seconds (s). Such hyperpolarization is mediated by the activation of two families of Ca2+-activated K+ channels. Big conductance (BK) channels contribute to AP repolarization, whereas small conductance (SK) channels underlie the AHP and mediate firing frequency and spike-frequency adaptation. Both fast AHPs (fAHP) and sAHPs were not observed during spontaneous firing of fast rhythmic APs. Small calcium-activated potassium current was recorded as a tail current by a depolarization step from −60 mV to 40 mV. Agonist activation of GnRH neurons caused a significant increase in sAHP that was partially sensitive to apamin. Also, treatment of GnRH neurons with 10 nM GnRH increased the occurrence of high-frequency broad APs, with unchanged decay constants for fAHP and mAHP. In contrast, treatment of GnRH neurons with 1 µM GnRH abolished mAHP current, but did not affect the occurrence of fAHP current. This was followed by subthreshold after-depolarization potential (ADP) and significant reduction of the AP firing frequency. These data indicate that AP- and GnRH-driven Ca2+influx in GnRH neurons determines the profile of AHP currents and consequently mediates firing frequency and the spike-profile. The majority of recorded GnRH neurons showed irregular AP firing with transition between slow, high spike amplitude tonic firing, and intervals of fast, lower spike-amplitude, burst-like AP firing. Transition from slow to fast tonic AP firing was associated with the appearance of switching APs that are characterized by fAHP, threshold afterdepolarization, and initiation of fast AP firing. Treatment of hypothalamic GnRH neurons with nanomolar GnRH concentrations increased the occurrence of fast tonic APs, and reduced the duration of mAHP current. In contrast, treatment of hypothalamic GnRH neurons with micromolar GnRH concentrations abolished the appearance of fast tonic AP firing and did not affect the occurrence of slow tonic AP firing. The inhibitory action of high GnRH concentrations on AP firing was prevented by PTX, and the underling currents were identified as GIRK currents. These responses indicate that agonist stimulation of endogenous GnRH receptors expressed in GnRH neurons activates GIRK channels, leading to suppression of membrane excitability and inhibition of AP firing. The data show that AP- and GnRH-driven calcium influx, and coupling of GnRH-R to Gi/o in GnRH neurons, determine the profile of after-hyperpolarization currents and consequently mediate firing frequency and the spike-profile. GnRH-induced modulation of calcium influx, and the consequent changes in AHP current, indicate that the plasma-membrane GnRH receptors expressed in hypothalamic GnRH neurons are important modulators of their neuronal excitability.
Role of CD74 in prostate cancer
Little is known about the protein–protein interactions that regulate the trafficking of the angiotensin II type I receptor (AGTR1) through the biosynthetic pathway. The membrane-proximal region of the cytoplasmic tail of the AGTR1 has been identified by site-directed mutagenesis studies as an essential site for normal AGTR1 folding and surface expression. Based on yeast two-hybrid screening of a human kidney cDNA library with the AGTR1 carboxyl-terminal tail as bait, we identified the invariant chain (CD74) as a novel interacting protein. This association was confirmed by co-immunoprecipitation and co-localization studies. The binding site for CD74 on the AGTR1 carboxyl-terminal tail was localized to a site previously identified as important for the exit of the AGTR1 from the endoplasmic reticulum (ER), and conserved in many G protein–coupled receptors. Transient co-expression of CD74 with the AGTR1 in CHO-K1 cells consistently reduced the AGTR1 density at the cell surface. Furthermore, the interaction of CD74 with the carboxy-terminal tail of the AGTR1 caused its retention in the ER and promoted its proteasomal degradation. These observations indicate that CD74 and the AGTR1 become associated in the early biosynthetic pathway and that CD74 is a negative regulator of AGTR1 expression.
There is emerging evidence that the incidence of cancer is reduced in patients undergoing long-term treatment with drugs that inhibit the renin-angiotensin system (RAS). ACE inhibitors have also been shown to inhibit tumor angiogenesis and tumor growth following the injection of sarcoma and fibrosarcoma cell lines in mice and to reduce lung metastasis following intravenous injection of Lewis lung carcinoma cells. Other evidence suggests that angiotensin II (Ang II) directly stimulates cell growth via the Ang II type 1 receptor (AT1-R) and that its blockade inhibits tumor growth. For instance, AT1-R antagonists suppress growth of human pancreatic cancer cells and, like ACE inhibitors, inhibit tumor angiogenesis and growth and lung metastasis resulting from injection of lung cancer cell lines. AT1-blockade also reduced tumor volume, vascular density, mitotic index and cell proliferation following injection of C6 glioma cells; in mice injected subcutaneously with B16-F1 melanoma, angiogenesis was prominent in wild-type but not in AT1-R deficient mice. Moreover, Ang II, acting via the AT1-R, enhanced the invasive potential and VEGF secretion in SKOV-3 ovarian cancer cells, and AT1-blockade resulted in a reduction in peritoneal dissemination, VEGF expression, and tumor angiogenesis. Moreover, in prostate cancer cell lines, AT1-blockade inhibits Ang II–mediated prostate cancer cell growth and and was shown to improve performance status and reduce prostate-specific antigen (PSA) levels in some patients with advanced hormone-refractory prostate cancer. While details of its physiological function require further investigation, it is clear that a locally based RAS exists in the prostate. There is a growing body of evidence that Ang II may have an important role in both normal prostatic growth and the development of a range of prostatic diseases, including benign prostatic hypertrophy and prostate cancer. Moreover, there is increasing evidence that drugs that target the RAS may be useful in the treatment of these conditions.
Ang II-induced ERK activation in bovine adrenal glomerulosa (BAG) cells
We previously analyzed Ang II–induced signaling in BAG cells to ascertain the extent and significance of ERK signaling and growth responses in a normal Ang II target tissue, the adrenal glomerulosa zone. These studies showed that Ang II caused rapid and transient activation and phosphorylation of ERK via the AT1 receptor. These actions were mediated by Gq, and to a lesser extent to Gi, and were associated with activation of PKC, Ras, and Raf-1 kinase. The activation of Raf-1 kinase by Ang II in BAG cells was dependent on Ras, and was mediated in part by Gi and PI 3-kinase. It has since become evident that ERK activation by Ang II and many other GPCR agonist is mediated by transactivation of the EGF receptor, as noted above. The BAG cell also expresses receptors for LPA, bradykinin, serotonin, glutamate, dopamine, and adrenergic agonists, so will be of value as a model for studying interactions between multiple GPCRs with a variety of signaling pathways arising from distinct G proteins and an array of actions on the secretory, growth, and proliferative activities of a native Ang II target cell.
Publications
- Krsmanovic LZ, Hu L, Leung P-K, Feng H, Catt KJ. The hypothalamic GnRH pulse generator: multiple regulatory mechanisms. Trends Endocrinol Metabol 2009 20:402-408.
- Szaszák M, Chen HD, Chen HC, Baukal A, Hunyady L, Catt KJ. Identification of the invariant chain (CD74) as an angiotensin AGTR1 receptor-interacting protein. J Endocrinol 2008 199:165-176.
- Chow L, Rezmann L, Catt KJ, Louis WJ, Frauman AG, Nahmias C, Louis SNS. Role of the renin-angiotensin system in prostate cancer. Mol Cell Endocrinol 2009 302:219-229.
- Yin X, Li B, Chen H, Catt KJ. Differential signaling pathways in angiotensin II- and epidermal growth factor signaling in LNcaP and PC3 cancer cells. Mol Pharmacol 2008 74:1223-1233.
- Krsmanovic LZ, Hu L, Leung P-K, Feng H, Catt KJ. Pulsatile GnRH secretion: roles of G protein-coupled receptors, second messengers and ion channels. Mol Cell Endocrinol 2009 [E-pub ahead of print].
Collaborators
- Hao-Chia Chen, PhD, Program in Developmental Endocrinology and Genetics, NICHD, Bethesda, MD
- Richard Hauger, MD, VA, University of California-San Diego, La Jolla, CA
- László Hunyady, MD, PhD, DSc, Semmelweis University of Medicine, Budapest, Hungary
- Simon Louis, PhD, University of Melbourne, Austin Health, Heidelberg, Victoria, Australia
- William Louis, MD, University of Melbourne, Austin Health, Heidelberg, Victoria, Australia
- Antonio Martínez-Fuentes, PhD, Universidad de Córdoba, Córdoba, Spain
- Nadia Mores, MD, Università Cattolica del Sacro Cuore, Rome, Italy
- J. Alberto Olivares-Reyes, PhD, Centro de Investigación y de Estudios Avanzados del Instituto Politécnico Nacional, Mexico City, Mexico
- Márta Szaszák, PhD, Institut für Medizinisch Mikrobiologie und Hygiene, Universität Lübeck, Lübeck, Germany
Contact
For further information, contact cattk@mail.nih.gov.