You are here: Home > Section on Cellular Differentiation
Molecular Genetics of Heritable Human Disorders
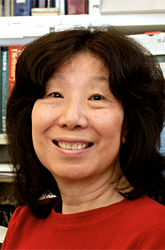
- Janice Y. Chou, PhD, Head, Section on Cellular Differentiation
- Hyun-Sik Jun, PhD, Postdoctoral Fellow
- Young Mol Lee, PhD, Visiting Fellow
- Su Ru Lin, PhD, Visiting Fellow
- Brian C. Mansfield, PhD, Guest Researcher
- Chi-Jiunn Pann, BS, Senior Research Assistant
- Matthew Lau, BS, Postbaccalaureate Fellow
Glycogen storage disease type I (GSD-I) consists of two subtypes: GSD-Ia, deficient in G6Pase-alpha (or G6PC), and GSD-Ib, deficient in the glucose-6-phosphate transporter (G6PT). A third disease, G6Pase-beta (or G6PC3) deficiency also groups with GSD-I. G6Pase-alpha and G6Pase-beta are endoplasmic reticulum (ER)–bound G6P hydrolases, with active sites lying inside the lumen, which depend upon G6PT to translocate G6P from the cytoplasm into the ER lumen. The G6PT/G6Pase-alpha complex maintains interprandial glucose homeostasis while the G6PT/G6Pase-beta complex maintains neutrophil homeostasis and function. GSD-Ia and GSD-Ib patients manifest a common metabolic phenotype of impaired glucose homeostasis not shared by G6Pase-beta deficiency. GSD-Ib and G6Pase-beta–deficient patients manifest a common myeloid phenotype of neutropenia and neutrophil dysfunction not shared by GSD-Ia. Recent studies reveal that neutrophils express the G6PT/G6Pase-beta complex to produce endogenous glucose. Inactivation of G6PT or G6Pase-beta leads to enhanced neutrophil apoptosis, which underlies neutropenia and neutrophil dysfunction in GSD-Ib and G6Pase-beta deficiency. There is no cure for GSD-I and G6PC3 deficiency. Animal models of the three disorders are available and are being exploited to both delineate the disease more precisely and develop new treatment approaches, including gene therapy.
Normoglycemia alone is insufficient to prevent hepatocellular adenoma, a long-term complication in GSD-Ib.
GSD-Ib patients develop the long-term complication of hepatocellular adenomas (HCA). To evaluate whether maintaining normoglycemia in GSD-Ib could prevent HCA, we infused neonatal GSD-Ib mice with adeno-associated virus (AAV) carrying G6PT and examined their metabolic and myeloid phenotypes for the 72-week study. The AAV vector delivered the G6PT transgene to the liver and bone marrow. Long-term metabolic correction was achieved alongside a transient myeloid correction. Hepatic G6PT activity was 50% of wild-type levels by 2 weeks after infusion but declined rapidly thereafter to reach 3% of wild-type levels by age 6 to 72 weeks. Despite this, the infused mice maintained normoglycemia throughout the study and exhibited near normal growth and normalized serum metabolite profiles. However, all five AAV-treated GSD-Ib mice that lived over 50 weeks accumulated excessive hepatic glycogen and fat. Two mice developed steatohepatitis and multiple HCAs with one undergoing malignant transformation. The results suggest that normoglycemia alone cannot prevent hepatic steatosis and glycogen accumulation or the development of HCAs in GSD-Ib, providing one explanation why GSD-Ib patients maintaining normoglycemia under intense dietary therapy continue to be at risk for this long-term complication.
Generation of mice with a conditional allele for G6pc
To improve our understanding of the roles of G6Pase-alpha (G6PC) in different tissues and in pathological conditions, we generated mice harboring a conditional null allele for G6pc by flanking exon 3 of the G6pc gene with loxP sites. We confirmed the null phenotype by using the EIIa-Cre transgenic approach to generate mice lacking exon 3 of the G6pc gene. The resulting homozygous Cre-recombined null mice manifest a phenotype mimicking G6Pase-alpha–deficient mice and human GSD-Ia patients. This G6pc conditional null allele will be valuable in the examination of the consequences of tissue-specific G6Pase-alpha deficiency and the mechanisms of long-term complications in GSD-Ia.
Lack of glucose recycling between ER and cytoplasm underlies cellular dysfunction in G6Pase-beta-deficient neutrophils in a congenital neutropenia syndrome.
G6PC3 deficiency, characterized by neutropenia and neutrophil dysfunction, is caused by deficiencies in G6Pase-beta (or G6PC3), which converts G6P into glucose, the primary energy source of neutrophils. Enhanced neutrophil endoplasmic reticulum (ER) stress and apoptosis underlie neutropenia in G6PC3 deficiency, but the precise functional role of G6Pase-beta in neutrophils remains unknown. We hypothesized that the ER recycles G6Pase-beta–generated glucose to the cytoplasm, thus regulating the amount of available cytoplasmic glucose/G6P in neutrophils. Accordingly, a G6Pase-beta deficiency would impair glycolysis and hexose monophosphate shunt activities, leading to reductions in lactate production, ATP production, and NADPH oxidase activity. Using non-apoptotic neutrophils, we showed that glucose transporter-1 translocation is impaired in neutrophils from G6pc3−/− mice and G6PC3-deficient patients along with impaired glucose uptake in G6pc3−/− neutrophils. Moreover, levels of G6P, lactate, and ATP are markedly lower in murine and human G6PC3-deficient neutrophils than in their respective controls. In parallel, the expression of NADPH oxidase subunits and membrane translocation of p47phox are down-regulated in murine and human G6PC3-deficient neutrophils. The results establish that, in non-apoptotic neutrophils, G6Pase-beta is essential for normal energy homeostasis. A G6Pase-beta deficiency prevents recycling of ER glucose to the cytoplasm, leading to neutrophil dysfunction.
Complete normalization of hepatic G6PC deficiency in murine GSD-Ia using gene therapy
GSD-Ia patients deficient in G6Pase-alpha (or G6PC) manifest impaired glucose homeostasis. We examined the efficacy of liver G6Pase-alpha delivery mediated by AAV-GPE, an AAV serotype 8 vector expressing human G6Pase-alpha directed by the human G6PC promoter/enhancer (GPE), and compared it with AAV-CBA, which directed murine G6Pase-alpha expression using a hybrid chicken beta-actin (CBA)promoter/CMV enhancer. The AAV-GPE–directed hepatic G6Pase-alpha expression in the infused G6pc−/− mice declined 12-fold from age 2 to 6 weeks but stabilized at wild-type levels from age 6 to 24 weeks. In contrast, the expression directed by AAV-CBA declined 95-fold over 24 weeks, demonstrating that the GPE is more effective in directing persistent in vivo hepatic transgene expression. We further showed that the rapid decline in transgene expression directed by AAV-CBA results from an inflammatory immune response elicited by the AAV-CBA vector. The AAV-GPE–treated G6pc−/− mice exhibit normal levels of blood glucose, blood metabolites, hepatic glycogen, and hepatic fat. Moreover the mice maintained normal blood glucose levels even after 6 hours of fasting. The complete normalization of hepatic G6Pase-alpha deficiency by the G6PC promoter/enhancer holds promise for the future of gene therapy in human GSD-Ia patients.
Oxidative stress mediates nephropathy in GSD-Ia.
GSD-Ia patients manifest impaired glucose homeostasis with the long-term complication of renal disease. We previously showed that renal fibrosis in GSD-Ia is mediated by the angiotensin/transforming growth factor-beta (TGF-beta1) pathway, which also elicits renal damage through oxidative stress. We have now elucidated the mechanism of renal disease by showing that renal expression of Nox-2, p22phox, and p47phox, components of NADPH oxidase, are up-regulated in GSD-Ia mice compared with controls. Akt/protein kinase B, a downstream mediator of angiotensin II and TGF-beta1, is also activated, leading to phosphorylation and inactivation of the Forkhead box O family of transcription factors. This in turn triggers down-regulation of superoxide dismutase and catalase activities, which play essential roles in oxidative detoxification in mammals. Renal oxidative stress in GSD-Ia mice is demonstrated by increased oxidation of dihydroethidium and by oxidative damage of DNA. Importantly, renal dysfunction, reflected in elevated serum levels of blood urea nitrogen, reduced renal catalase activity, and increased renal fibrosis, is improved in GSD-Ia mice treated with the antioxidant drug tempol. These data provide the first evidence that oxidative stress is one of the mechanisms underlying GSD-Ia nephropathy.
Publications
- Yiu WH, Pan CJ, Mead PA, Starost MF, Mansfield BC, Chou JY. Normoglycemia alone is insufficient to prevent long term complications of hepatocellular adenoma in glycogen storage disease type Ib mice. J Hepatol 2009;51:909-917.
- Chou JY, Jun HS, Mansfield BC. Neutropenia in type Ib glycogen storage disease. Curr Opin Hematol 2010;17:36-42.
- Yiu WH, Mead PA, Jun HS, Mansfield BC, Chou JY. Oxidative stress mediates nephropathy in type Ia glycogen storage disease. Lab Invest 2010;90:620-629.
- Yiu WH, Lee YM, Peng WT, Pan C-J, Mead PA, Mansfield BC, Chou JY. Complete Normalization of Hepatic G6PC Deficiency in Murine Glycogen Storage Disease Type Ia using Gene Therapy. Mol Ther 2010;18:1076-1084.
- Jun HS, Lee YM, McDermott DH, DeRavin SS, Murphy PM, Mansfield BC, Chou JY. Lack of glucose recycling between endoplasmic reticulum and cytoplasm underlies cellular dysfunction in glucose-6-phosphatase-beta-deficient neutrophils in a congenital neutropenia syndrome. Blood 2010;May 24. [Epub ahead of print].
Collaborators
- Eric J. Lee, DVM, Program on Genomics of Differentiation, NICHD, Bethesda, MD
- Matthew F. Starost, PhD, DVR, Division of Veterinary Resources, NIH, Bethesda, MD
- David A. Weinstein, MD, University of Florida College of Medicine, Gainesville, FL
- Heiner Westphal, MD, Program on Genomics of Differentiation, NICHD, Bethesda, MD
- David H. McDermott, MD, Laboratory of Host Defenses, NIAID, Bethesda, MD
- Philip M. Murphy, MD, Laboratory of Host Defenses, NIAID, Bethesda, MD
- Suk See DeRavin, MD, Laboratory of Host Defenses, NIAID, Bethesda, MD
Contact
For further information, contact chouja@mail.nih.gov.