You are here: Home > Section on Cellular and Synaptic Physiology
Hippocampal Interneurons and Their Role in the Control of Network Excitability

- Chris J. McBain, PhD, Head, Section on Cellular and Synaptic Physiology
- Kenneth Pelkey, PhD, Staff Scientist
- Ramesh Chittajulla, PhD, Senior Research Fellow
- Elizabeth Barksdale, PhD, Postdoctoral Fellow
- Sean Barron, PhD, Postdoctoral Fellow
- Megan Wyeth, PhD, Postdoctoral Fellow
- Jose Matta, PhD, Visiting Fellow
- Brian Jeffries, BS, Biologist
- Xiaoqing Yuan, MSc, Biologist
- Madhav Sukumaran, BSc, Graduate Student
- Geoff Vargish, BS, Graduate Student
- Carla Lopez, BS, NIH Academy
Cortical and hippocampal local circuit GABAergic inhibitory interneurons are "tailor-made" to control Na+- and Ca2+-dependent action potential generation, to regulate synaptic transmission and plasticity, and to pace large-scale synchronous oscillatory activity. The axons of this diverse cell population make local, usually short-range projections (some subpopulations project their axons over considerable distances) and release the inhibitory neurotransmitter gamma-aminobutyric acid (GABA) onto a variety of targets. A mounting appreciation of the roles played by interneurons in several mental health conditions such as epilepsy, stroke, Alzheimer's disease, and schizophrenia have placed this important cell type center stage in cortical circuit research. Our main objective is to understand how both ionic and synaptic mechanisms regulate the activity of local-circuit GABAergic interneurons and principal neurons at the level of small well-defined networks. To this end, we use a variety of electrophysiological, immunohistochemical, molecular, and genetic approaches in both wild-type and transgenic animals. Over the past five years, we have continued our study of the differential mechanisms of glutamatergic and GABAergic synaptic transmission and plasticity within the hippocampal formation and the modulation of voltage- and ligand-gated channels expressed in inhibitory neurons. We have also incorporated genetic approaches to unravel the embryogenesis and development of hippocampal interneurons and the circuits in which they are embedded.
Neto1 is an auxiliary subunit of native synaptic kainate receptors.
Although auxiliary proteins have been identified for AMPA and NMDA receptor complexes, little is known about kainate receptor (KAR) complex proteins. In collaboration with Mike Salter's and Rod McInnis' laboratories, Ken Pelkey showed that Neto1 is an auxiliary subunit for endogenous synaptic KARs. We found that Neto1 and KARs co-immunoprecipitated from brain lysates, from postsynaptic densities (PSDs) and, in a manner dependent on Neto1 CUB domains, when co-expressed in heterologous cells. In Neto1-null mice, we observed about a 50% reduction in the abundance of GluK2-KARs in hippocampal PSDs. Neto1 strongly localized to the CA3 stratum lucidum, and loss of Neto1 resulted in a selective deficit in KAR–mediated neurotransmission at mossy fiber (MF)–CA3 synapses. KAR–mediated excitatory post-synaptic currents (EPSCs) in Neto1-null mice had lower amplitudes and decayed more rapidly than did those in wild-type mice. In contrast, loss of Neto2, which also localizes to the stratum lucidum and interacts with KARs, had no effect on KAR synaptic abundance or MF–CA3 transmission. Indeed, MF–CA3 KAR deficits in Neto1/Neto2 double-null mutant mice were indistinguishable from Neto1 single-null mice. Thus, our findings establish Neto1 as an auxiliary protein required for synaptic function of KARs. The ability of Neto1 to regulate both NMDA receptors and KARs reveals a unique dual role in controlling synaptic transmission by serving as an auxiliary protein for these two classes of ionotropic glutamate receptors in a synapse-specific fashion.
A follow up study is currently under way using electron microscopy to determine the precise distribution of kainate receptor subunits at the mossy fiber synapse and how their expression and number is influenced by the various NETO subunits.
A blueprint for the spatiotemporal origins of mouse hippocampal interneuron diversity
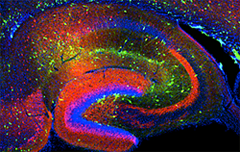
Click image to enlarge.
Figure 1. Interneurons in the GAD-65 GFP mouse
Numerous subpopulations are present within a hippocampal section derived from a GAD-65 GFP transgenic mouse.
Although vastly outnumbered by other neurons, inhibitory interneurons critically pace and synchronize excitatory principal cell populations to coordinate cortical information processing. Precision in this control relies upon a remarkable diversity of interneurons primarily determined during embryogenesis by genetic restriction of neuronal potential at the progenitor stage. Like their neocortical counterparts, hippocampal interneurons arise from medial and caudal ganglionic eminence (MGE and CGE) precursors. However, while studies of the early specification of neocortical interneurons are rapidly advancing, similar lineage analyses of hippocampal interneurons have lagged. A "hippocampocentric" investigation is necessary, given that several hippocampal interneuron subtypes remain poorly represented in the neocortical literature. Thus, we investigated the spatiotemporal origins of hippocampal interneurons using transgenic mice that specifically report GFP expression in MGE– and CGE–derived interneurons either constitutively or inducibly. We found that hippocampal interneurons are produced in two neurogenic waves between embryonic days E9–E12 and E12–E16 from the MGE and CGE, respectively, and invade the hippocampus by E14. In the mature hippocampus, CGE–derived interneurons primarily localize to superficial layers in the strata lacunosum moleculare and deep radiatum, while MGE–derived interneurons readily populate all layers with preference for the strata pyramidale and oriens. Combined molecular, anatomical, and electrophysiological interrogation of MGE/CGE–derived interneurons revealed that MGE produces parvalbumin-, somatostatin-, and nitric oxide synthase–expressing interneurons that include fast-spiking basket, bistratified, axo-axonic, oriens-lacunosum moleculare, neurogliaform, and ivy cells. In contrast, CGE–derived interneurons contain cholecystokinin, calretinin, vasoactive intestinal peptide, and reelin and include non-fast-spiking basket, Schaffer collateral–associated, mossy fiber–associated, trilaminar, and additional neurogliaform cells. Our findings provide a basic blueprint of the developmental origins of hippocampal interneuron diversity.
Young dentate granule cells mediate pattern separation, whereas old granule cells facilitate pattern completion.
Adult-born granule cells (GCs), a minor population of cells in the hippocampal dentate gyrus, are highly active during the first few weeks after functional integration into the neuronal network, distinguishing them from less active, older adult-born GCs and the major population of dentate GCs generated developmentally. To ascertain whether young and old GCs perform distinct memory functions, we created a transgenic mouse in which output of old GCs was specifically inhibited while leaving a substantial portion of young GCs intact. The mice exhibited enhanced or normal pattern separation between similar contexts, which was curtailed following ablation of young GCs. Furthermore, the mutant mice exhibited deficits in rapid pattern completion. Therefore, pattern separation requires adult-born young GCs but not old GCs whereas older GCs contribute to the rapid recall by pattern completion. Our data suggest that as adult-born GCs age, their function switches from pattern separation to rapid pattern completion.
Developmental origin dictates interneuron AMPA and NMDA receptor subunit composition and plasticity.
Disrupted excitatory synapse maturation in cortical GABAergic interneurons can precipitate an imbalance in circuit excitation/inhibition that is posited to underlie the progression of neuropsychiatric disorders such as schizophrenia. However, establishing the basic developmental programs for nascent synapses in GABAergic cells is confounded by their sparsity, heterogeneity, and late postnatal acquisition of subtype-defining characteristics. To overcome these hurdles, we investigated synaptic properties of individual interneurons throughout early postnatal development, targeting cells based on lineage from MGE or CGE progenitors. This approach enabled us to interrogate non-overlapping interneuron populations whose fates can be predicted from origin. We found stereotypical developmental differences in synaptic AMPA and NMDA receptors between MGE– and CGE–derived interneurons including differential GluN2 subunit composition of NMDARs and expression of Ca2+-permeable AMPARs. Our findings establish ganglionic eminence–dependent rules for early postnatal synaptic integration programs of distinct interneuron cohorts including parvalbumin and cholecystokinin-expressing basket cells.
Additional Funding
- Former Visiting Fellow Dr. Christine Torborg was the recipient of a Pharmacology Research Associate (PRAT) Fellowship.
- Dr. Matta was the recipient of a Pharmacology Research Associate (PRAT) Fellowship.
Publications
- Tricoire L, Pelkey KA, Daw MI, Sousa VH, Miyoshi G, Jeffries B, Cauli B, Fishell G, McBain CJ. Common origins of hippocampal Ivy and nitric oxide synthase expressing neurogliaform cells. J Neurosci 2010;30:2165-2176.
- Cea-del Rio CA, Lawrence JJ, Tricoire L, Erdelyi F, Szabo G, McBain CJ. M3 muscarinic acetylcholine receptor expression confers differential cholinergic modulation to neurochemically distinct hippocampal basket cell subtypes. J Neurosci 2010;30:6011-6024.
- Tang M, Pelkey KA, Ng D, Ivakine E, McBain CJ, Salter MW, McInnes RR. Neto1 is an auxiliary subunit of native synaptic kainate receptors. J Neurosci 2011;31:10009-10018.
- Tricoire L, Pelkey KA, Erkkila BE, Jeffries BG, Yuan XQ, McBain CJ. A blueprint for the spatiotemporal origins of hippocampal interneuron diversity. J Neurosci 2011;31:10948-10970.
- Nakashiba T, Cushman JD, Pelkey KA, Renaudineau S, Buhl DL, McHugh TJ, Rodriguez Barrera V, Chittajallu R, Iwamoto KS, McBain CJ, Fanselow MS, Tonegawa S. Young dentate granule cells mediate pattern separation, whereas old granule cells facilitate pattern completion. Cell 2012;149:188-201.
Collaborators
- Gordon Fishell, PhD, New York University, New York, NY
- Roderick McInnes, PhD, Lady Davis Research Institute, McGill University, Toronto, Canada
- Michael Salter, PhD, Centre for the Study of Pain, Hospital for Sick Children, Toronto, Canada
- Susumo Tonegawa, PhD, Picower Institute for Learning and Memory, MIT, Cambridge, MA
- Katalin Toth, PhD, Université Laval, Quebec City, Canada
- Paul Worley, PhD, The Johns Hopkins University, Baltimore, MD
Contact
For more information, email mcbainc@mail.nih.gov or visit neuroscience.nih.gov/Faculty/Profile/chris-mcbain.aspx.