You are here: Home > Section on Molecular Neurobiology
Mechanisms Regulating Activity-Dependent Synaptic Plasticity and Gene Expression
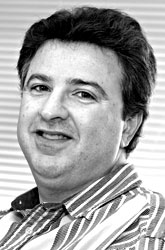
- Andres Buonanno, PhD, Head, Section on Molecular Neurobiology
- Detlef Vullhorst, PhD, Staff Scientist
- Irina Karavanova, PhD, Biologist
- Elias Leiva-Salcedo, PhD, Visiting Fellow
- Megan J. Janssen, PhD, Intramural Research Training Award Fellow
- Robert Mitchell, PhD, Intramural Research Training Award Fellow
A key feature in the etiology of several developmental psychiatric disorders such as schizophrenia, autism, and ADHD is the failure of cortical microcircuits to properly regulate the balance between excitation and inhibition. This balance is important to synchronize the firing pattern of local neuron ensembles. Such network activity, in particular oscillatory activity in the gamma-frequency range (30–80 Hz), is altered in psychiatric disorders and may account for the cognitive and behavioral symptoms. The Section on Molecular Neurobiology (SMN) is interested in how Neuregulin and its receptor ErbB4, which are both genetically linked to psychiatric disorders, function in the developing brain to regulate synaptic plasticity, neuronal network activity, and behaviors that, in rodents, model features of psychiatric disorders. We identified a functional interaction between Neuregulin/ErbB4 and dopamine signaling in GABAergic interneurons that is critical for understanding how Neuregulin can regulate excitation/inhibition balance and synchronous activity in neuronal networks, both processes being important for cognitive functions altered in psychiatric disorders.
To understand how Neuregulin-ErbB4 signaling regulates synaptic plasticity, neuronal network activity, and behaviors associated with psychiatric disorders, we are investigating how Neuregulin mediates its effects either indirectly by modulating excitation/inhibitory balance in neural circuits or directly by regulating the synaptic and intrinsic properties of ErbB4-expressing cells. To achieve these goals, we are using a combination of techniques, including electrophysiological recordings in acute slices and dissociated cultures prepared from normal and genetically altered mice, reverse-microdialysis neurochemistry, confocal fluorescence microscopy in fixed and live tissue, proteomics analyses, and behavioral testing. The ultimate goal of this multi-disciplinary approach is to generate holistic models in which to investigate the developmental impact of genes that modulate excitatory/inhibitory balance and neuronal network activity and that consequently affect behaviors and cognitive functions altered in psychiatric disorders.
Conservation—from rodents to primates—of ErbB4 expression in GABAergic interneurons in the hippocampus and cortex
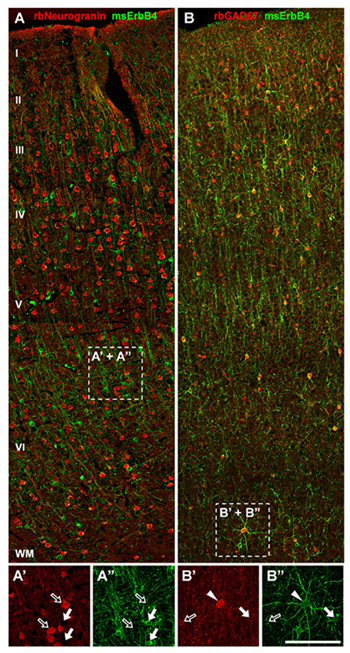
Click image to enlarge.
Figure 1. ErbB4 is expressed in a subset of GABAergic interneurons in monkey frontal cortex.
A, A', A": ErbB4 immunoreactivity (arrows) is absent from pyramidal cells labeled with rabbit anti-neurogranin antibody (open arrows). B, B', B": ErbB4 is strongly expressed in GAD-67–positive interneurons in layers II to VI, similar to rodent and human frontal cortex. Cells co-expressing both markers are labeled with arrowheads.
Knowledge of the cellular and subcellular localization of ErbB4 is important for understanding how Neuregulin (NRG) regulates neuronal network activity and behavior. In collaboration with Ludovic Tricore and Chris McBain, we demonstrated, using a combination of single-cell RT–PCR and electrophysiological profiling in mice, that ErbB4 transcripts are expressed GABAergic interneurons and not in excitatory pyramidal cells. This finding was confirmed with specific monoclonal ErbB4 antibodies generated in our lab, which showed that the receptor accumulates in the somato-dendritic region of cortical GABAergic interneurons in rodents, rhesus monkeys, and humans; presynaptic or axonal expression of ErbB4 was rarely detected. In collaboration with David Lewis' group, we found that, in primate cortex, parvalbumin-, calretinin-, and cholecystokinin-positive GABAergic interneurons express ErbB4, but that calbindin-positive cells do not (1). This cross-species conservation of cell type–specific and subcellular ErbB4 expression pattern validates the use of rodents to analyze cellular and neural circuit effects of abnormal ErbB4 function as a means to model endophenotypes pertinent to psychiatric disorders (Figure 1).
Importance of Neuregulin/ErbB4 signaling in parvalbumin-positive fast-spiking interneurons
We previously showed, using reverse-microdialysis neurochemistry and electrophysiology, that NRG1 signaling via ErbB4 potently triggers dopamine release in the hippocampus and reverses LTP at Schaeffer collateral-to-CA1 (SC–CA1) synapses by activating dopamine D4 receptors. Importantly, we found that ErbB4 is not detectable in excitatory pyramidal neurons but is abundantly expressed in GABAergic interneurons, particularly in fast-spiking parvalbumin-positive basket cells, observations that strongly suggested indirect effects of NRG/ErbB4 on glutamatergic synaptic plasticity. To address this question directly, we compared the effects of full vs. parvalbumin (PV) interneuron–specific Erbb4 ablation in genetically engineered mice. As expected, NRG–mediated inhibition of LTP induction and reversal of early-phase LTP were lacking in full ErbB4 knockout mice. Interestingly, ErbB4 ablation in PV interneurons was sufficient to reproduce the effects of the full ErbB4 knockout. We also compared the two ErbB4 mutant mouse strains in a battery of behavioral tests relevant to psychiatric disorders and found that PV–restricted mutants replicated many, albeit not all, abnormalities observed in full ErbB4 mutant mice. The findings highlight the role of PV interneurons as a critical nexus of NRG/ErbB4 signaling and provide further evidence in support of a possible contribution of this pathway, genetically linked with increased risk for schizophrenia, to the pathophysiology underlying psychiatric disorders (2) (Figure 2).
Figure 2. LTP in ErbB4 and NRG-1 mutant mice
Compared with normal controls (black circles in A,B,D,E), slices from ErbB4−/− (A: solid blue circles) and ErbB4−/+ mice (B: open blue circles) exhibited enhanced LTP following pairing. C: Top, Western blot analysis of ErbB4 protein in whole tissue lysates from frontal cortex (FC) and hippocampus (HC) of control ErbB4 f/f (−Cre) and PV-Cre;ErbB4 (+Cre) mice. Representative results from two animals are shown for each genotype. Bottom, co-immunofluorescence of ErbB4 (red) and Pv (green) in the frontal cortex. In ErbB4 f/f controls, numerous PV+ cells coexpress ErbB4 (arrows). In contrast, ErbB4 is not detected in PV+ cells from PV–Cre;ErbB4 mice. Arrowheads mark ErbB4+ cells that are negative for PV. D: Selective ablation of ErbB4 in PV interneurons (green) results in higher LTP. E: LTP was also increased in slices from NRG-1+/− mice (grey).
NRG/ErbB4 and dopamine D4 receptor signaling converge in PV neurons and regulate gamma oscillations.
We previously reported that NRG/ErbB4 signaling modulates the power of kainate-induced hippocampal gamma oscillations. Because NRG1 dramatically increases extracellular dopamine levels in the hippocampus, we investigated the relationship between NRG/ErbB and dopamine signaling in hippocampal gamma oscillations. In collaboration with André Fisahn's lab, we used selective agonists for different dopamine G-coupled receptors that enhance (D1 and D5 receptors) or suppress (D2, D3 and D4 receptors) the synthesis of cAMP, and found that only an agonist specific for D4 receptors (PD168077) augmented the power of gamma oscillations. By contrast, agonists for D1/D5 and D2R/D3 receptors were without effect. The increase in gamma oscillation power induced by PD168077 was entirely blocked by a highly specific D4 receptor antagonist (L-745,870), further stressing the importance of this receptor for neuronal network activity. Importantly, we found that clozapine, an antipsychotic that preferentially targets D4 receptors, and L-745,870 also blocked increases in gamma oscillation power by NRG1. Using double in situ hybridization (in collaboration with Ursula Winzer-Serhan) and immunofluorescence histochemistry, we showed that hippocampal D4 receptor mRNA and protein are highest in a subset of GABAergic interneurons. Importantly, D4 and ErbB4 receptors are coexpressed in PV+ fast-spiking basket cells, a type of somatic-targeting GABAergic interneuron that is critically important for regulating gamma oscillations (3). The novel cross-talk between D4 and ErbB4 receptor signaling to augment gamma oscillation power and their coexpression in PV-positive interneurons suggest a cellular mechanism that may be compromised in various psychiatric disorders affecting cognitive control (Figure 3).
Figure 3. Convergence of ErbB4 and D4R signaling in PV–positive interneurons
Representative sample traces (left) and power spectra (right) of kainate-induced gamma oscillations in rat slices. NRG-1b (2 nM) was tested in combination with (A) kainate alone (n=8), (B) kainate and L-745,870 (50 nM; n=8), or (C) kainate and clozapine (2 µM; n=5). D: Summary analysis of normalized data from (A–C). Values for kainate treatment are set as 100%. E–H: Representative images of triple immunofluorescence histochemistry in hippocampal CA3. Overlay images showing PV+ neurons that also coexpress D4 and ErbB4 receptors (arrows).
Neuregulin directly decreases voltage-gated sodium currents in hippocampal ErbB4–expresssing interneurons.
One of our primary goals has been to investigate whether and how NRG1 directly affects the intrinsic properties of ErbB4–positive (ErbB4+) interneurons. To this end, we resorted to using dissociated hippocampal cultures, which are devoid of all hippocampal-projecting afferent connections, and to blocking all synaptic activity pharmacologically. We initially analyzed the effects of NRG1 on the excitability of ErbB4+ and ErbB4− neurons, identified in live labeling by an ectodomain-targeting antibody, using whole-cell current-clamp recordings. We found that NRG1 decreased firing of ErbB4+ but not ErbB4− neurons by shifting the action potential threshold. Using voltage-clamp recordings, we determined that these effects are primarily attributable to decreased voltage-gated sodium channel activity, as current density was attenuated by about 60% after 20 minutes of NRG1 treatment. This is the first study to identify direct actions of NRG1 on voltage-gated sodium channel function in ErbB4–expressing interneurons, thereby offering novel insights into how NRG1/ErbB4 signaling can directly impact GABAergic interneuron activity and potentially affect excitatory/inhibitory balance (4) (Figures 4 and 5).
Figure 4. NRG1 reduces ErbB4 neuron excitability.
A: Neurons live-labeled with an ErbB4 antibody (arrow: ErbB4+; arrowhead: ErbB4−). B: Representative traces of current injection steps in ErbB4+ or ErbB4− neurons treated with NRG1 (E+N and E−N), vehicle-treated ErbB4+ neurons (E+V) and ErbB4+ neurons treated with NRG1 and the ErbB receptor inhibitor PD158780 (E+NPD). The rheobase current is shown in red and one subsequent trace is shown in black. Current was injected in 50 pA steps. C: Summary graph of normalized current threshold to evoke an AP at 20 min. D: Summary graph of normalized inter-spike interval (ISI) at 20 min averaged over all current. Inset shows example of an ErbB4 cell firing at baseline (black) and 20 min (red) after NRG1 application at 700 pA injection.
Figure 5. NRG1 application dramatically reduces voltage-gated sodium channel currents.
A, B: Representative traces of Nav currents from an ErbB4 interneuron before (baseline) and after NRG1 (A) or vehicle (B) application for 10 and 20 min. Far right shows overlaid maximum Na currents. Inset shows voltage-clamp protocol used to evoke somatic Na channel current. C, D: Averaged I/V relationship for Nav channel activation showing the NRG1–specific decrease in Na channel density (C) compared with vehicle (D). E: Individual Na channel current responses to NRG1 (E+N, left) or vehicle application (E+V, right) at 20 min are plotted with their own baseline (gray lines). Average response depicted with black line. F: Averaged normalized maximum current density from E+N and E+V cells.
NRG1 signaling directly influences AMPA receptor (AMPAR) activity in glutamatergic ErbB4–expressing cerebellar granule neurons.
Previous studies demonstrated how NRG1 indirectly affects plasticity at glutamatergic synapses in principal glutamatergic neurons in the hippocampus and frontal cortex. In collaboration with Catherine Fenster, we analyzed the effects of NRG1 on developing cultured cerebellar granule cells (CGCs). The cultures consist predominantly of granule neurons that express ErbB4. We found that, under baseline conditions of CGCs grown for 10-12 days in vitro, NRG1 does not affect whole-cell AMPAR– or NMDAR–mediated currents nor the frequency or amplitude of spontaneous NMDAR– or AMPAR–mediated miniature excitatory post-synaptic currents. However, we found that, in CGCs, high glycine induces a form of chemical potentiation (chemLTP) characterized by an increase in AMPAR mEPSC frequency that is reduced by NRG1 treatment. Because in our culture conditions CGCs express very low levels of the AMPAR GluA1 subunit but high levels of GluA4, our data suggest that the NRG1 effect could be mediated by GluA4 subunits. The study shows, for the first time, that high glycine can induce plasticity at glutamatergic synapses in CGCs and that acute NRG1/ErbB signaling can regulate glutamatergic plasticity in CGCs. Taken together with those from our previous studies, our results suggest that, similar to SC-CA1 synapses, NRG1 effects on CGCs are activity-dependent and mediated by modulation of synaptic AMPARs (5) (Figure 6).
Figure 6. NRG1 reduces AMPAR–mEPSC frequency, but not amplitude, following chemLTP.
A: Experimental protocol used to assess effects of treatments following high glycine. Cells were immediately voltage-clamped following exposure to high glycine, and baseline data were collected for 4–6 min prior to and following treatment onset with either PD158780, NRG1, or vehicle control. Cells were continuously perfused with extracellular fluid (ECF) containing 1 mM MgCl2, 500 nM tetrodotoxin, and 50 µM bicuculline. Data points plot mean AMPA–mEPSC amplitude (B) and frequency (C) at baseline and at indicated time points following treatments. **P < 0.001 (repeated measures ANOVA, time × treatment interaction).
Proteomics approach to understanding NRG/ErbB4–proximal signaling targets
In order to discover potentially novel and direct biological targets of NRG/ErbB4, we are employing, in collaboration with Sanford Markey's group, an unbiased liquid chromatography–tandem mass spectrometry (LC/MS/MS) proteomics approach to determine the composition of the ErbB4 receptor proteome. We recently reported validation of our receptor monoclonal antibody for affinity purification of ErbB4 complexes and their analysis by LC/MS/MS. In recent experiments, we used a similar method to isolate endogenous ErbB4 complexes from metabolically active synaptosomes following NRG-1 stimulation. Using this approach, we discovered that NRG/ErbB4 signaling alters the ion channel composition of cellular microdomains associated with ErbB4. Our goal is to determine the functional consequences of these alterations.
Publications
- Neddens J, Fish KN, Tricoire L, Vullhorst D, Shamir A, Chung W, Lewis DA, McBain CJ, Buonanno A. Conserved interneuron-specific ErbB4 expression in frontal cortex of rodents, monkeys, and humans: implications for schizophrenia. Biol Psychiatry 2011;70:636-645.
- Shamir A, Kwon OB, Karavanova I, Vullhorst D, Leiva-Salcedo E, Janssen MJ, Buonanno A. The importance of the NRG-1/ErbB4 pathway for synaptic plasticity and behaviors associated with psychiatric disorders. J Neurosci 2012;32:2988-2897.
- Andersson RH, Johnston A, Herman PA, Winzer-Serhan UH, Karavanova I, Vullhorst D, Fisahn A, Buonanno A. Neuregulin and dopamine modulation of hippocampal gamma oscillations is dependent on dopamine D4 receptors. Proc Natl Acad Sci USA 2012;109:13118-13123.
- Janssen-Schroeder MJ, Leiva Salcedo DE, Buonanno A. Neuregulin directly increases voltage-gated sodium currents in hippocampal ErbB4-expressing interneurons. J Neurosci 2012;32:13889-13895.
- Fenster C, Vullhorst D, Buonanno A. Acute neuregulin-1 signaling influences AMPA receptor mediated responses in cultured cerebellar granule neurons. Brain Res Bull 2012;87:21-29.
Collaborators
- Jacqueline N. Crawley, PhD, Laboratory of Behavioral Neuroscience, NIMH, Bethesda, MD
- Shashank Dravid, PhD, Creighton University, Omaha, NE
- Catherine Fenster, PhD, The College of Wooster, Wooster, OH
- André Fisahn, PhD, Karolinska Institute, Stockholm, Sweden
- Kenneth Fish, PhD, University of Pittsburgh, Pittsburgh, PA
- Kristian Gundersen, PhD, University of Oslo, Oslo, Norway
- Luis Hernández, MD, Universidad de los Andes, Mérida, Venezuela
- David A. Lewis, MD, University of Pittsburgh, Pittsburgh, PA
- John E. Lisman, PhD, Brandeis University, Waltham, MA
- Sanford P. Markey, PhD, Laboratory of Neurotoxicology, NIMH, Bethesda, MD
- Christopher McBain, PhD, Program on Developmental Neuroscience, NICHD, Bethesda, MD
- Jörg Neddens, PhD, JSW Life Sciences, Grambach, Austria
- Zaheer Rana, PhD, University of Oslo, Oslo, Norway
- Ludovic Tricore, PhD, Program on Developmental Neuroscience, NICHD, Bethesda, MD
- Ursula H. Winzer-Serhan, PhD, Texas A&M University, College Station, TX
Contact
For more information, email buonanno@mail.nih.gov or visit smn.nichd.nih.gov.