You are here: Home > Molecular Neurophysiology and Biophysics Section
Potassium Channels and Dendritic Function in Hippocampal Pyramidal Neurons
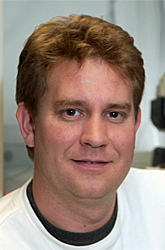
- Dax Hoffman, PhD, Head, Molecular Neurophysiology and Biophysics Section
- Lin Lin, PhD, Research Fellow
- Erin Gray, PhD, Postdoctoral Fellow
- Eun-young Kim, PhD, Postdoctoral Fellow
- Michael W. Nestor, PhD, Postdoctoral Fellow
- Emilie Campanac, PhD, Visiting Fellow
- Begum Choudhury, MS, Biologist
- Laura Long, BA, Postbaccalaureate Fellow
- Ben Throesch, BS, Postbaccalaureate Fellow
- Wei Sun, BS, Graduate Student
The central nervous system underlies all our experiences, actions, emotions, knowledge, and memories. With billions of neurons each firing hundreds of times per second, the complexity of the brain is stunning. To pare down the task of understanding something so complex, our research approach calls for studying the workings of a single central neuron—the pyramidal neuron from the CA1 region of the hippocampus. The hippocampus is essential for long-term memory in humans and is among the first brain regions affected by epilepsy and Alzheimer's disease. To understand how the hippocampus stores and processes information, we focus on one of its principal cell types, the CA1 pyramidal neuron. Each pyramidal neuron in the CA1 region of the hippocampus receives tens of thousands of inputs onto its dendrites, and it is commonly thought that information is stored by altering the strength of individual synapses (synaptic plasticity). Recent evidence suggests that the regulation of synaptic surface expression of glutamate receptors can, in part, determine synaptic strength. However, the dendrites contain an abundance of ion channels that are involved in receiving, transforming, and relaying information in the dendrites, adding an additional layer of complexity to neuronal information processing.
We found that the A-type potassium channel subunit Kv4.2 is highly expressed in the dendritic regions of CA1 neurons in the hippocampus and, as one of the primary regulators of dendritic excitability, plays a pivotal role in information processing. Kv4.2 is targeted for modulation during the types of plasticity thought to underlie learning and memory. Moreover, the functional expression level of Kv4.2 was found to regulate the subtype expression of NMDA–type glutamate receptors, the predominate molecular devices controlling synaptic plasticity and memory. We are currently following up on these findings with more detailed investigations into the mechanisms of activity-dependent Kv4.2 regulation. In addition, we have begun investigating the role of dendritic voltage-gated channels in CNS disorders, including autism-spectrum disorder and Alzheimer's disease, that exhibit profound changes in neuronal excitability along with learning and memory deficits.
Voltage-gated ion channel expression and trafficking in dendrites
Kv4.2 control of firing patterns in hippocampal CA1 pyramidal neurons: Although recent molecular cloning studies found that several families of voltage-gated K+ channel genes are expressed in the mammalian brain, information regarding the relationship between the protein products of these genes and their various neuronal functions is, at present, lacking. Our lab used a combination of molecular, electrophysiological, and imaging techniques to show that the A-type voltage-gated potassium channel subunit Kv4.2 controls action potential (AP) half-width, frequency-dependent AP broadening, and dendritic AP propagation. More recently, we examined the role of A-type K+ channels in regulating intrinsic excitability of CA1 pyramidal neurons of the hippocampus after synapse-specific long-term potentiation (LTP) induction (a potential form of cellular memory). Using electrophysiological recordings in nucleated patches from organotypic slices of rat hippocampus, we found that LTP induced potentiation of excitability, which was accompanied by a two-phased change in A-type K+ channel activity. Induction of LTP resulted in an immediate but short-lasting hyperpolarization of the voltage-dependence of steady-state A-type K+ channel inactivation, along with a progressive, long-lasting reduction in peak A-current density. Blocking clathrin-mediated endocytosis prevented the A-current reduction and most measures of intrinsic plasticity. The results suggest that two temporally distinct but overlapping mechanisms of A-channel downregulation together contribute to the plasticity of intrinsic excitability. The change in intrinsic plasticity resulted in a global enhancement of excitatory postsynaptic potential–spike coupling.
Kv4.2 trafficking in CA1 pyramidal neuron dendrites: We previously reported that neuronal stimulation results in a redistribution of Kv4.2 channels away from dendritic spines to the dendritic shaft. The activity-dependent redistribution of Kv4.2 required activation of NMDA–type glutamate receptors and calcium influx, two requirements shared with synaptic plasticity, which, as already mentioned, is thought to underlie learning and memory. Given the nonuniform distribution of Kv4.2 channels in CA1 dendrites, Mike Nestor performed experiments to test the hypothesis that Kv4.2 channels are differentially trafficked at different regions along the apical dendrite during basal activity and upon stimulation in CA1 neurons (1). Proximal (50-150 μm from the soma, primary and oblique dendrites) and distal (>200 μm) apical dendrites were selected. We used the fluorescence recovery after photobleaching (FRAP) technique to measure basal cycling rates of EGFP-tagged Kv4.2 (Kv4.2g). We found that the cycling rate of Kv4.2 channels was one order of magnitude slower at both primary and oblique dendrites between 50-150 μm from the soma than the cycling rate of those at at 200-250 μm from the soma, whereas Kv4.2 channel cycling rose significantly at 200-250 μm. Expression of a Kv4.2 mutant (Kv4.2gS552A), which lacks a phosphorylation site for protein kinase-A (PKA), abolished the distance-dependent change in channel cycling, demonstrating that phosphorylation by PKA underlies the elevated mobility in distal dendrites. Neuronal stimulation increased cycling of Kv4.2 channels significantly at distal sites only. The activity-dependent increase in Kv4.2 cycling at distal dendrites was blocked by expression of Kv4.2gS552A. The results indicate that distance-dependent Kv4.2 mobility is regulated by activity-dependent phosphorylation of Kv4.2 by PKA.
Lin Lin discovered a potential source of PKA modulation of Kv4.2 when she identified A-kinase–anchoring proteins (AKAPs) as novel accessory subunits for Kv4.2 (2). AKAPs target PKA to glutamate receptor and ion channel complexes to allow for discrete, local signaling. We determined that the C-terminal domain of Kv4.2 interacts with an internal region of AKAP79/150 that overlaps with its MAGUK binding domain. AKAP79/150–anchored PKA activity was shown to control Kv4.2 surface expression in heterologous cells and hippocampal neurons. Consistent with these findings, disrupting PKA anchoring leads to a reduction in neuronal excitability, whereas preventing dephosphorylation by the phosphatase calcineurin results in increased excitability. The results demonstrate that AKAP79/150 provides a platform for dynamic PKA regulation of Kv4.2 expression, thus fundamentally impacting neuronal excitability.
Laura Long collaborates with Juan Bonifacino's lab (NICHD) to investigate whether and how clathrin-associated adaptor protein (AP) complexes play a role in the subcellular protein trafficking of voltage-gated potassium channel Kv4.2. Using co-immunoprecipitation and yeast in situ hybridization assays, we found binding between Kv4.2 and APs. We are currently determining the binding sites and investigating the impact of their interaction on the subcellular distribution and trafficking of Kv4.2.
Voltage-gated calcium channel trafficking: Erin Gray began a research project investigating activity-dependent trafficking of another important dendritically localized channel, the voltage-gated calcium channel Cav2.3. Cav2.3 generates large calcium spikes selectively in dendritic spines, which shape both synaptic potentials and back-propagating action potentials. Furthermore, modulation of these channels by activation of kinases such as PKC and CamKII can dramatically affect synaptic plasticity and neuronal excitability. Gray cloned Cav2.3 into expression plasmids to generate Cav2.3 with a GFP tag on its N- or C-terminus. After confirming the functional expression of all three constructs in HEK cells, she expressed the recombinant channels in dissociated hippocampal neuron culture. She will begin imaging studies to determine the localization and activity-dependent trafficking of Cav2.3 in neurons.
Role of voltage-gated ion channels in synaptic development and plasticity
Neuronal activity is critical for the formation and modification of neural circuits during brain development. In hippocampal CA1 pyramidal dendrites, A-type voltage-gated K+ currents control excitability. We have found that altering functional Kv4.2 expression level leads to a rapid, bidirectional remodeling of CA1 synapses. Neurons exhibiting enhanced A-type K+ current showed a lower ratio of synaptic NR2B to NR2A subunits and did not exhibit LTP. Conversely, reducing A-current by expression of a Kv4.2 dominant negative or through genomic knockout of Kv4.2 led to a higher ratio of NR2B to NR2A subunits and enhanced LTP. Our data suggest that A-type K+ channels are an integral part of a synaptic complex that regulates Ca2+ signaling through spontaneous NMDA receptor activation to control synaptic NMDA receptor expression and plasticity.
Eun-young Kim used Kv4.2 knock-out (Kv4.2–KO) mice along with acute in vivo expression of Kv4.2 or its dominant negative pore mutant to examine the role of Kv4.2 in the development of CA1 synapses (3). Kv4.2 expression induced synaptic maturation in juvenile wild-type (WT) mice and rescued developmentally delayed synapses in adult Kv4.2–KO mice. Furthermore, NMDA–type glutamate receptor subunit composition could be restored to the juvenile form in WT adult synapses by functionally down-regulating Kv4.2 levels. The results suggest that Kv4.2 regulation of excitability determines synaptic maturation state, which can be bidirectionally adjusted into adulthood.
The dipeptidyl peptidase-like protein 6 (DPP6) is an auxiliary subunit for Kv4 A-type K+ channels that, in addition to enhancing channel surface expression, potently accelerates their activation, inactivation, and recovery from inactivation. DPP6 is structurally remarkable for its large extracellular domain, the function of which is unknown. DPP6 is roughly 50% similar to CD26, a member of this family, binds to components of the extracellular matrix like collagen, fibronectin, and integrin β1, and mediates roles in cell adhesion, cellular trafficking, and T cell activation; it also has a cysteine-rich domain that binds to components of the extracellular matrix. Therefore, DPP6 may play a similar role to that of CD26. This past year, Lin Lin examined the role of DPP6 in neuronal development. DPP6 RNAi knockdown and genetic deletion demonstrated DPP6 involvement in the formation and stability of a dendritic filopodia during early neuronal development. We found that DPP6 induced Rho-family small GTPase Cdc42 activation, which typically leads to the formation of filopodia by organizing the actin cytoskeleton. As a consequence of the reducion in filopodia, hippocampal neurons lacking DPP6 showed a sparser dendritic branching pattern along with fewer spines throughout development and into adulthood compared with WT neurons. Electrophysiological and imaging experiments showed that these deficits lead to a reduction in functional synapses. Our findings demonstrate that DPP6 plays an important developmental role in dendrite branching, spine maturation, and synaptogenesis in hippocampal CA1 pyramidal neurons, with potential clinical implications.
Dendritic excitability and disease
Discovering the etiology of many central nervous system (CNS) disorders has proven elusive because susceptibility to these diseases can be a product of multiple factors such as genetics, epigenetics, and environment. Advances in molecular biology and wide-scale genomics have shown that many heterogeneous genetic mutations may contribute to neuronal pathologies and behaviors observed in CNS disorders. Despite this complex range of genetic and physiological factors, many disorders of the CNS display common dysfunctions in memory. We proposed that mechanisms underlying the development of many CNS disorders share an underlying cause involving abnormal dendritic integration of synaptic signals (4). By understanding the relationship between molecular genetics and dendritic computation, future research may uncover important links between neuronal physiology at the cellular level and higher-order circuit and network abnormalities observed in CNS disorders and illuminate their subsequent effect on memory.
The Kv4 auxiliary protein DPP6 protein was recently identified in large copy-number variants screens from some populations as an Autism Spectrum Disorder and amyotrophic lateral sclerosis (ALS) target gene. DPP6 enhances the opening probability of Kv4 channels and enhances channel surface expression in heterologous systems. In dendritic recordings from DPP6–KO mice, Wei Sun discovered that DPP6 is critical for generating the A-type K+ current gradient observed in CA1 dendrites. The loss this gradient led to hyper-excitable dendrites, with implications for information storage and coding (5).
We previously demonstrated a role of A-type K+ channels in regulating the intrinsic excitability of CA1 pyramidal neurons of the hippocampus after the induction of synaptic plasticity. Such intrinsic plasticity might represent an information storage mechanism available to neurons in addition to synaptic plasticity. Ben Throesch studied the excitability and intrinsic plasticity of CA1 hippocampal pyramidal neuron apical dendrites in mouse models for Fragile X Syndrome and Alzheimer's disease.
Intrinsic plasticity has also been observed after drug addiction. The addictive drug cocaine's psychostimulant effects are attributed to inhibition of the dopamine transporter, which raises dopaminergic transmission. Chronic exposure to cocaine leads to neurodaptations in several membrane conductances of neurons localized in the medial prefrontal cortex (mPCF) and nucleus accumbens. Most studies have focused on layer 5 pyramidal neurons (the circuit output) while the response of local GABAergic interneurons to cocaine remains poorly understood. Emilie Campanac recorded from fast-spiking interneurons (FS-INs) after repeated cocaine exposure and found altered membrane excitability. She also observed a reduction in miniature excitatory postsynaptic currents but no effect on miniature inhibitory postsynaptic current activity. In animals with a history of cocaine exposure, dopamine receptor D2 activation is less effective in increasing FS-INs intrinsic excitability than in animals not exposed to the drug. Interestingly, these alterations are not observed until a week or more after the last cocaine exposure, suggesting that the dampening of D2R mediated response can be a compensatory mechanism to rein in the excitability of FS-INs.
Publications
- Nestor MW, Hoffman DA. Differential cycling rates of Kv4.2 channels in proximal and distal dendrites of hippocampal CA1 pyramidal neurons. Hippocampus 2012;22(5):969-980.
- Lin L, Sun W, Kung F, Dell'Acqua ML, Hoffman DA. AKAP79/150 impacts intrinsic excitability of hippocampal neurons through phospho-regulation of A-type K+ channel trafficking. J Neurosci 2011;26;31(4):1323-1332.
- Kim E-Y, Hoffman DA. Dynamic regulation of synaptic maturation state by voltage-gated A-type K+ channels in CA1 hippocampal pyramidal neurons. J Neurosci 2012;in press.
- Nestor MW, Hoffman DA. Aberrant dendritic excitability: a common pathophysiology in CNS disorders affecting memory? Mol Neurobiol 2012;45(3):478-487.
- Sun W, Lin L, Maffie, JK, Petralia, RS, Rudy B, Hoffman DA. DPP6 establishes the A-type K+ current gradient critical for the regulation of dendritic excitability in CA1 hippocampal neurons. Neuron 2011;71(6):1102-1115.
Collaborator
- Mark Dell'Acqua, PhD, University of Colorado Denver, Denver, CO
- Johannes Hell, PhD, University of California Davis, Davis, CA
- Andrew Holmes, PhD, Laboratory for Integrative Neuroscience, NIAAA, Rockville, MD
- Bernardo Rudy, MD, PhD, NYU Medical Center, New York, NY
- Constantine A. Stratakis, MD, D(Med)Sc, Program in Developmental Endocrinology and Genetics, NICHD, Bethesda, MD
Contact
For more information, email buonanno@mail.nih.gov or visit neuroscience.nih.gov/Faculty/Profile/andres-buonanno.aspx.